Asia Pacific J Clin Nutr (1997) 6(1): 49-55

Adipose
tissue expansion and the development of
obesity: influence of dietary fat type
DB Hausman PhD, MY Loh BS, WP Flatt PhD and RJ Martin PhD
Department of Foods and Nutrition,
University of Georgia, Athens, Georgia, USA
Recent studies indicate that the prevalence of obesity
in adults has increased by 30% or more in the past decade, with
increases in both genders and in all ethnic and racial populations
and age groups. Obesity is associated with many chronic diseases
and alterations in physiologic function including cardiovascular
disease, hypertension, diabetes mellitus, gallbladder disease and
certain types of cancer. Much attention regarding dietary influences
on obesity development or prevention has focused on high fat diets.
Many studies have confirmed that high fat feeding leads to an expansion
of adipose tissue mass through an increase in fat cell size and/or
number and to the subsequent development of obesity. However, there
is little definitive information on the effect of type of dietary
fat, especially palm oil, on adipose tissue cellularity and the
development of obesity. These studies were designed to determine
whether dietary fat of different sources vary in their ability to
produce obesity and to begin to elucidate the mechanism by which
such divergence occurs. Male Osborne-Mendel rats were fed either
a low fat (15% calories) or one of three high fat diets (65% calories)
for 12 weeks. The predominant fat source in the high fat diets was
either soybean oil, tallow, or palm-olein (a fraction of palm oil).
Final body weight was not influenced by fat level or type; however,
percent carcass lipid and fat pad weight were higher in soybean
oil and tallow fed rats than in low fat and palm-olein fed rats.
Fat pad specific increases in cell size and cell number were observed
for tallow and soybean oil fed compared to low fat and palm-olein
fed rats. Serum triglycerides were higher in the tallow and palm-olein
fed rats compared to low fat fed rats; no significant effects of
dietary fat type on serum cholesterol were observed. These results
indicate that palm-olein, unlike tallow and soybean oil, were comparable
to a low fat diet concerning fat pad weight, body composition and
adipose tissue cellularity when fed for twelve weeks as 65% of energy
intake. The lower fat storage in the palm-olein fed rats is perhaps
associated with a slower rate of triglyceride uptake and/ or a reduced
fat cell proliferative capacity. The influence of dietary fat type
on the proliferative capacity of the pre-adipocytes and on the production
of a local or systemic adipogenic factor is being determined in
subsequent studies.
Key words: Palm oil, fat cell sizes,
fat cell number, rats
Introduction
Obesity is associated with many chronic
diseases and alterations in physiologic function including cardiovascular
disease, hypertension, diabetes mellitus, gallbladder disease
and certain types of cancer1. Obesity is a major public
health problem in the United States and Europe and is becoming increasingly
important in many other areas of the world2. The prevalence
of obesity in adults in the U. S. has increased by 30% or more in
the past decade, with increases in both genders and in all ethnic
and racial populations and age groups3. It is now estimated
that over one third of the adult population in the US are obese3.
Obesity is likewise very common in Europe, with a prevalence of considerably
greater than 10% based on ninetieth percentile body mass indices4.
Recent studies indicate a strong correlation between the increasing
prevalence of obesity and diet-related chronic diseases in many developing
countries including China, Pacific Island populations and Brazil5-7.
The etiology of human obesity is quite complex, involving
genetic, metabolic, behavioral and environmental factors. Although
obesity is believed to have a strong genetic component8,
the increased incidence of obesity in specific population groups undergoing
Westernization indicates the importance of dietary and lifestyle changes
in the manifestation of this disease5,6. Among dietary
factors, both total energy intake and fat intake are significantly
correlated with body mass index in these population groups5.
However, increased intake of fat energy is associated with a greater
per unit increase in body mass than is increased intake of energy
from non-fat sources. Therefore, much attention regarding dietary
influences on obesity development or prevention has focused on high
fat diets.
Many animal studies have confirmed that high fat feeding
leads to an expansion of adipose tissue mass through an increase in
fat cell size and/or number and to the subsequent development of obesity9-15.
Hyperphagia9,10 and decreased energy expenditure (via changes
in diet induced thermogenesis)11 are believed to be contributing
factors to the development of high fat diet induced obesity, while
changes in adipose tissue cellularity influence the reversibility
of this condition12,13. It is thought that once the peak
capacity for storing lipid is reached in high fat fed animals, increases
in fat cell number are triggered12. These changes in fat
cell number are permanent, as substitution of the high fat diet with
a low fat diet leads to a reduction in body weight and fat cell size
but not in fat cell number12,13. Recent evidence indicates
that changes in adipose cellularity during the development of obesity
in high fat fed rats may be associated with the appearance of a locally
produced factor(s) capable of stimulating adipose cell proliferation16.
There is little definitive information on the effect
of type of dietary fat on adipose tissue cellularity and the development
of obesity. Alterations in dietary fat type have been shown to influence
membrane composition, function and metabolic processes in many tissues17-27.
Kirtland et al14 observed that long-term feeding
of high fat diets (20%) containing beef drippings vs. maize oil had
no influence on body weight gain or adipose tissue cellularity in
guinea pigs. More recently, Shimomura et al28 reported
a decreased accumulation of body fat in rats fed safflower oil vs.
beef tallow associated with similar weight gain throughout the dietary
treatment. In contrast, Pan et al29 observed a decreased
weight gain with long-term feeding of high fat diets containing tallow
as compared to safflower oil or olive oil. The effect of diet on total
body lipid composition was not determined in that study. Both Su and
Jones30 and Hill et al31, reported a
reduction in energy accumulation in rats fed fish oil but no differences
in energy accumulation between rats fed the other dietary fat types
(olive oil vs. beef tallow and lard vs. corn oil, respectively). Shillabeer
and Lau32, reported differences in body and fat pad weights
and in fat cell diameter between rats fed different high fat diets
as compared to chow-fed rats; however, differences between rats fed
various types of dietary fat (beef tallow, safflower oil) were observed
only when the diets were fed at restricted levels.
Long-term studies of the effect of different types
of dietary fats on the development of obesity have generally not included
palm oil, one of the worlds most widely consumed oils33.
Palm oil is a partially saturated oil containing equal amounts of
saturated and unsaturated fatty acids. Palm-olein, a modified fraction
of palm oil, contains more unsaturated than saturated fatty acids.
Recent research indicates that palm oil, unlike other partially saturated
dietary fats, does not raise plasma cholesterol levels34-36.
Palm oil has also been shown to decrease tumor development during
cancer-induction in several animal studies35-37. Although
much research has been done on the potential beneficial effects of
palm oil on serum lipids and tumor suppression, there is little information
concerning the relationship between palm oil consumption and obesity,
another major chronic disease. A few studies have reported no differences
in final body weight17,38,39 epididymal fat pad weight17
or fat cell diameter17 between rats fed palm oil as compared
to several other types of dietary fats; however these studies were
all of fairly short duration (four weeks or less). This study was
undertaken therefore, to determine the effect of longer-term feeding
of diets containing high levels of various types of dietary fat, including
palm-olein, on lipid accretion and adipose cellularity in Osborne-Mendel
rats. In subsequent studies we hope to discern whether variations
in lipid accretion associated with the various types of dietary fat
are due to alterations in endogenous production of local adipogenic
factors.
Methods
Twenty-seven male Osborne-Mendel rats (125g
body weight) were purchased from the Genetic Resource Division of
NIH (Washington, DC, USA). After a three-day acclimatization period,
rats were randomly allocated to one of four diet groups: Low Fat,
Soybean Oil, Tallow, or Palm-Olein. The low fat diet contained 15%
calories as fat, whereas the three high fat diets contained 65% calories
as fat (Table 1). All diets contained 20% calories as protein and
adequate vitamins and minerals. Non-lipid diet ingredients were purchased
from United States Biomedical Co. (Cleveland, OH; USA). Source and
approximate fatty acid composition of fats and oils used in diet formulation
are presented in Table 2. Diets were prepared weekly, stored at 4°
C and fed ad libitum. Body weight and food intake measurements
were recorded at least three times weekly. Spillage was taken into
account. As problems were experienced with the acceptance of the somewhat
liquid high fat diets, the initial diet formulation was modified after
one week. Fiber content of the high fat diets was increased to 17.9
g/ 100 g (Table 1). Rats remained on the reformulated diets for an
additional eleven weeks. Data from the compositional analysis of diet
samples (via bomb calorimetry at the Poultry Research Laboratory,
Dept Poultry Sci, University of Georgia, Athens, GA; USA) taken during
week seven of the study are also given in Table 1.
During week seven on the study, total daily energy
expenditure was determined using a computer controlled indirect calorimeter
(OXYMAX, Columbus, Instruments, Columbus, OH; USA). Ten automated
open circuit respiration chambers were used to measure air flow using
a mass flow controller, carbon dioxide concentrations using an infrared
gas analyzer and oxygen concentration using an Oxymax sensor battery.
Chamber temperatures were also recorded throughout each 24-hour monitoring
period. Average oxygen consumption, average daily carbon dioxide production,
respiratory quotient and average heat production were subsequently
determined.
Table 1. Composition of the experimental diets.
|
Low fat
|
High fats oybean oil
|
High fat tallow
|
High fat palm-olein
|
|
g/ 100 g diet
|
Casein |
19.5
|
27.5
|
27.5
|
27.5
|
Cornstarch |
53.6
|
17.5
|
17.5
|
17.5
|
Sucrose |
10.0
|
3.2
|
3.2
|
3.2
|
Fat: |
|
|
|
|
Soybean
oil |
6.85
|
41.8
|
6.8
|
6.8
|
Other |
------
|
------
|
35.0
|
35.0
|
Fiber |
5.0
|
5.0 (17.9)*
|
5.0 (17.9)*
|
5.0 (17.9)*
|
Minerals |
3.5
|
3.5
|
3.5
|
3.5
|
Vitamins |
1.0
|
1.0
|
1.0
|
1.0
|
L-Cysteine |
0.3
|
0.3
|
0.3
|
0.3
|
Choline
chloride |
0.25
|
0.25
|
0.25
|
0.25
|
Caloric
density** (cal/ g) |
4389
|
5941
|
6191
|
6028
|
% dry matter** |
93.7
|
97.7
|
97.4
|
97.4
|
*After 1 week on feeding, high fat diets were reformulated
so as to improve texture and acceptability. Fiber was the only component
altered. Revised values for fiber and caloric density are given in
parenthesis.
AIN-76 mineral and vitamin mixtures (AIN 1977).
**Compositional analysis of diet samples via bomb calorimetry (Poultry
Research Laboratory, Dept Poultry Sci, University of Georgia).
After twelve weeks on the experimental diets, rats
were killed by decapitation between 0900 and 1100 hours. Blood was
collected, stored on ice and centrifuged at 2500 x g for 30 minutes
to obtain serum. Serum was stored at -20° C until used for assay of:
total cholesterol (Cholesterol 20 kit #352-20; Sigma Chemical Co,
St Louis, MO; USA), triglycerides (Triglyceride-Int kit #336-10; Sigma
Chemical Co, St Louis, MO; USA), glucose (Glucose Trinder kit #315-10;
Sigma Chemical Co, St Louis, MO; USA) and insulin (125I-Insulin
ImmuChem radioimmunoassay kit; ICN Biomedicals, Costa Mesa, CA; USA).
Table 2. Fatty acid composition of the oils
and fats used in the experimental diets.
|
Soybean oil*
|
Tallow
|
Palm-olein§
|
|
(% of total fatty acids)
|
Saturated
|
14
|
50
|
46
|
Monounsaturated |
25
|
35.7
|
43
|
Polyunsaturated |
60
|
14.3
|
11
|
Values based on product specifications and compositional
analysis data furnished with individual products. *ICN
Biochemicals (Cleveland, OH); Wilsey A/V Fry Shortening
(City of Industry, CA); §Fuji Vegetable Oil, Inc (Savannah,
GA)
Inguinal, retroperitoneal and epididymal fat pads
were dissected completely and weighed. Triplicate samples of each
pad (40-60 mg) were taken for cell size and number analysis. The samples
were rinsed thoroughly with isotonic saline, weighed and fixed with
osmium tetroxide in 50 mM collidine-HCl buffer as described by Cartwright40,
prior to electronic counting on a Coulter ZM particle analyzer according
to the method of Hirsch and Gallian41. Use of this technique
allowed determination of both cell size distribution and total fat
pad cell number.
The remainder of the fat pads were returned to the
carcass. The carcass (minus the GI tract) was stored for subsequent
determination of carcass composition according to the method of Harris
and Martin42. Briefly, frozen carcasses were autoclaved
in individual sealed beakers for one hour at 121°C. When cool, each
carcass was ground in a blender with water. The slurry was homogenized
and samples of the homogenate taken for water, ash and fat analyses.
Water content was determined by the difference in weight of the triplicate
aliquots of the homogenate before and after drying to a constant weight
(85°C for 48 hours). Ash was analyzed by subsequent ashing of the
same samples in a furnace at 600°C for 12 hours. Additional aliquots
of homogenate were analyzed for lipid content. Lipid was determined
gravimetrically after extraction of the homogenate with chloroform:methanol
and evaporation of the extract to a constant weight. Protein was estimated
by subtracting the weight of lipid and ash from that of the dry matter.
Statistical
Analysis
The effect of diet treatment on food
intake, body weight gain, energy expenditure, body composition, and
adipose tissue cellularity was determined on a personal computer using
the SuperANOVA program (Abacus Concepts, Berkeley, CA; USA). Comparison
between the means was accomplished using the Least Squares Means procedure43.
Differences were considered statistically significant at the p <
0.05 level.
Results
As shown in Table 3, body weight gain throughout
the twelve week period of ad libitum feeding was unaffected
by either the level or type of dietary fat. Cumulative food intake,
on a per gram basis, was significantly greater in the low fat fed
group as compared to the three high fat fed groups. However, after
correction for differences in the caloric density of the diets (given
in Table 1) total caloric consumption was significantly greater in
the low fat and high fat tallow fed groups as compared to the high
fat soybean oil and high fat palm-olein fed groups. Digestibility
determinations conducted during week seven of the feeding trial indicated
a significantly higher digestiblity of the low fat diet (90.1 ± 0.4%
digestible dry matter) as compared to the three high fat diets (80.1
± 0.5, 78.0 ± 1.5 and 78.7 ± 0.9% digestible dry matter for the soybean
oil, tallow and palm-olein diets, respectively). Corrections for differences
in digestibility of the various diets resulted in a greater estimated
digestible energy intake for the twelve week dietary period for the
low fat fed group as compared to the high fat fed groups. Among the
high fat fed groups, the estimated digestible energy intake was significantly
higher for the tallow fed as compared to the soybean oil and palm-olein
fed rats.
Table 3. Food intake and body weight gain of
Osborne-Mendel rats fed a low fat diet or one of three high fat diets
for 12 weeks.
|
Low-fat
|
High fats oybean oil
|
High fat tallow
|
High fat palm-olein
|
Initial
body weight (g) |
141 ± 3.8
|
138 ± 3.5
|
138 ± 3.5
|
137.5 ± 3.5
|
Body weight
gain (g) |
299 ± 11
|
322 ± 10.2
|
315 ± 10.2
|
306 ± 10.2
|
Total food
intake (g) |
1781 ± 39a
|
1193 ± 36b
|
1310 ± 36b
|
1181± 36b
|
Total food
intake (Kcal) |
7816 ± 215a
|
7086 ± 199b
|
8112 ± 199a
|
7120 ± 199b
|
Estimated
digestible energy (Kcal) |
7042 ± 176a
|
5678 ± 163b
|
6323 ± 163c
|
5605 ± 163b
|
Values represent least squares
mean ± SEM for 6-7 rats per diet group. Estimated digestible energy
intake for the 12 week dietary period is based on digestible dry matter
determinations of 90.10, 80.12, 77.95 and 78.73% for the low fat,
soybean oil, tallow and palm-olein diets, respectively. Values within
a row with different superscripts are significantly different (p <
0.05).
Indirect calorimetry for determination of total daily
energy expenditure during week seven of the study indicated no significant
effect of level or type of dietary fat on daily oxygen consumption,
CO2 production or heat production (Table 4). As expected,
the respiratory quotient was significantly higher for the low fat
fed rats as compared to the three high fat fed groups. However, there
were no differences in respiratory quotient among the three high fat
fed groups.
Table 4. Energy expenditure
parameters of Osborne-Mendel rats fed a low fat diet or one of three
high fat diets.
|
Low-fat
|
High fat soybean oil
|
High fat tallow
|
High fat palm-olein
|
Oxygen consumption
(Liter/ day) |
10.1 ± 0.76
|
10.7 ± 0.7
|
10.1 ± 0.7
|
10.6 ± 0.7
|
CO2
production (Liter/ day) |
9.4 ± 0.7
|
8.4 ± 0.66
|
7.7 ± 0.66
|
8.3 ± 0.66
|
CO2/
O2 (Respiratory Quotient) |
0.93 ± 0.16a
|
0.78 ± 0.15b
|
0.76 ± 0.15b
|
0.78 ± 0.15b
|
Heat production
(kcal/ day ) |
50 ± 3.8
|
51 ± 3.5
|
48 ± 3.5
|
51 ± 3.5
|
Measurements were made over two
24-h periods during week seven of the study. Values represent least
squares means ± SEM for 6-7 rats per diet group. Values within a row
with different superscripts are significantly different (p < 0.05).
Despite the minimal effects of diet on body weight
gain, food intake and energy expenditure, significant differences
in carcass composition were observed between the dietary groups (Table
5). An approximate 25-34% increase in carcass lipid was observed in
rats fed the high fat soybean oil and tallow diets as compared to
those fed the low fat diet. However, percent carcass lipid was not
increased in the rats consuming the high fat palm-olein diet for twelve
weeks. Carcass protein was similar in all four dietary groups. Percent
carcass ash was lower in the rats fed the high soybean oil diet as
compared to the other three dietary groups. Percent carcass water
was significantly higher in the high fat palm-olein fed rats as compared
to rats fed the other two high fat diets.
Table 5. Body composition
of Osborne-Mendel rats fed a low fat diet or one of three high fat
diets for 12 weeks.
|
Low-fat
|
High fat soybean oil
|
High fat tallow
|
High fat palm-olein
|
Carcass
weight (g) |
400 ± 11
|
413 ± 9.8
|
407 ± 9.8
|
398 ± 9.8
|
Protein
(%) |
17.5 ± 0.7
|
16.3 ± 0.65
|
17.4 ± 0.65
|
16.6 ± 0.65
|
Lipid (%) |
11.3 ± 0.79a
|
15.1 ± 0.73b
|
14.3 ± 0.73b
|
11.6 ± 0.73a
|
Ash (%) |
1.72 ± 0.16a
|
1.13 ± 0.15b
|
1.42 ± 0.15a
|
1.43 ± 0.15a
|
Water (%) |
69.4 ± 0.93a,b
|
67.5 ± 0.86a
|
67.1 ± 0.86a
|
70.4 ± 0.86b
|
Values represent least squares
means ± SEM for 6-7 rats per diet group. Values within a row with
different superscripts are significantly different (p < 0.05).
Consistent with effects of dietary treatment on total
carcass lipid, significantly greater weights of the inguinal, epididymal
and retroperitoneal fat pads were observed in rats fed the high fat
soybean oil and tallow diets as compared to those fed the low fat
or the high fat palm-olein diets (Table 6). Inguinal fat pad weight
was increased by 41 and 27% respectively for the high fat soybean
oil and tallow fed rats as compared to the low fat fed group. Weights
of the epididymal and retroperitoneal fat pads were likewise increased
(by 49-57%) in rats fed the high fat soybean oil and tallow diets
as compared to the low fat fed controls. The increased fat pad weights
were associated with changes in both cell size and number. In the
inguinal fat pad, a significant increase in total fat cell number
was observed in rats fed the high fat soybean oil diet as compared
to the low fat fed controls. This increase was attributed to a greater
number of cells in both the smallest (20-30 mm)
and in several larger (80-100 and 100-140 mm) size ranges (Figure 1). Average cell size
in the inguinal fat pad was greatest for the high fat tallow fed group;
however, this was not found to be statistically significant (Table
6). Epididymal fat cell number tended to be increased in the rats
fed the high fat soybean oil and tallow diets as compared to the low
fat fed controls (P=0.06 and 0.053, respectively). As for the inguinal
fat pad, the numbers of cells in both the smallest (<40 mm) and in the 80-140 mm
size ranges were greater in the epididymal fat pads of the high fat
soybean oil and tallow fed rats as compared to the those of the low
fat fed animals (data not shown). In this fat pad, average cell volume
was significantly lower in rats fed the high fat palm-olein diet as
compared to those fed the low fat or high fat soybean oil diets. No
apparent differences in cell number relative to diet-ary treatments
were observed for the retroperitoneal fat pad. However, average fat
cell size was found to be significantly smaller in retroperitoneal
fat pads from the high palm-olein fed rats as compared to those of
the other high fat fed groups.
Table 6. Fat pad weights and cellularity parameters
of Osborne-Mendel rats fed a low fat diet or one of three high fat
diets for 12 weeks.
|
Low-fat
|
High fat soybean oil
|
High fat tallow
|
High fat palm-olein
|
Inguinal
fat pad |
|
|
|
|
Fat pad
weight (g) |
7.35 ± 0.61a
|
10.42 ± 0.56b
|
9.36 ± 0.56b
|
7.71 ± 0.56a
|
Cells /pad
(x 106) |
18.90 ± 3.11a
|
30.73 ± 2.88b
|
22.90 ± 2.88ab
|
19.08 ± 2.88a
|
Average
cell volume (pl) |
153 ± 16.1
|
160 ± 14.9
|
178 ± 14.9
|
135 ± 14.9
|
Epididymal
fat pad |
|
|
|
|
Fat pad
weight (g) |
6.83 ± 0.78a
|
10.11 ± 0.72b
|
10.47 ± 0.72b
|
7.96 ± 0.72a
|
Cells /pad
(x 106) |
16.39 ± 3.31
|
25.27 ± 3.06
|
25.58 ± 3.06
|
22.54 ± 3.06
|
Average
cell volume (pl) |
220 ± 12.2a
|
238 ± 11.3a
|
215.5 ± 11.3ab
|
184 ± 11.3b
|
Retroperitoneal
fat pad |
|
|
|
|
Fat pad
weight (g) |
5.83 ± 0.88a
|
9.14 ± 0.81b
|
8.69 ± 0.81b
|
5.96 ± 0.81a
|
Cells /pad
(x 106) |
18.15 ± 3.37
|
22.09 ± 2.85
|
14.17 ± 3.07
|
13.60 ± 3.07
|
Average
cell volume (pl) |
204 ± 16.7ab
|
246 ± 14.1a
|
234.9 ± 12.0a
|
178 ± 16.4b
|
Values represent least squares means ± SEM for 6-7
rats per diet group. Values within a row with different superscripts
are significantly different (p < 0.05).
As shown in Table 7, total serum cholesterol concentrations
were significantly lower in Osborne-Mendel rats fed the low fat diet
for twelve weeks as compared to those fed any of the high fat diets.
Serum cholesterol concentrations did not differ according to type
of fat fed in the high fat diets. Serum triglycerides were increased
approximately two-fold in rats fed the high fat tallow and palm-olein
diets as compared to those fed the low fat diet. Serum glucose and
insulin concentrations were not influenced by the level or type of
fat fed during this twelve-week study.
Table 7. Serum metabolite and insulin concentrations
of Osborne-Mendel rats fed a low fat diet or one of three high fat
diets.
|
Low-fat
|
High fat soybean oil
|
High fat tallow
|
High fat palm-olein
|
Total cholesterol
(mg/dL) |
71 ± 6.3a
|
90 ± 5.9b
|
94 ± 5.9b
|
89 ± 5.9b
|
Triglycerides
(mg/dL) |
66 ± 12.33a
|
93 ± 11.4a,b
|
23 ± 11.4b
|
124 ± 11.4b
|
Glucose
(mg/ dL) |
126 ± 5.6
|
126 ± 5.2
|
141 ± 5.2
|
143 ± 5.2
|
Insulin
(mU/ mL) |
42 ± 9.5
|
46 ± 8.8
|
28 ± 8.8
|
34 ± 8.8
|
Values represent least squares means ± SEM for 6-7
rats per diet group. Values within a row with different superscripts
are significantly different (p < 0.05).
Figure 1. Cell size distribution in inguinal
fat pad of Osborne-Mendel rats fed a low fat diet or one of three
high fat diets for 12 weeks. Samples of adipose tissue were fixed
in osmium tetroxide and cell size and number were deter-mined by Coulter
Counter analysis. Values are least squares means ± SEM for 6-7 rats
per diet groups. A significantly greater number of cells were observed
in the 20-30 mm and 80-140 mm size ranges for the rats fed the
high fat soybean oil diet as compared to the low fat fed controls
(P < 0.05).
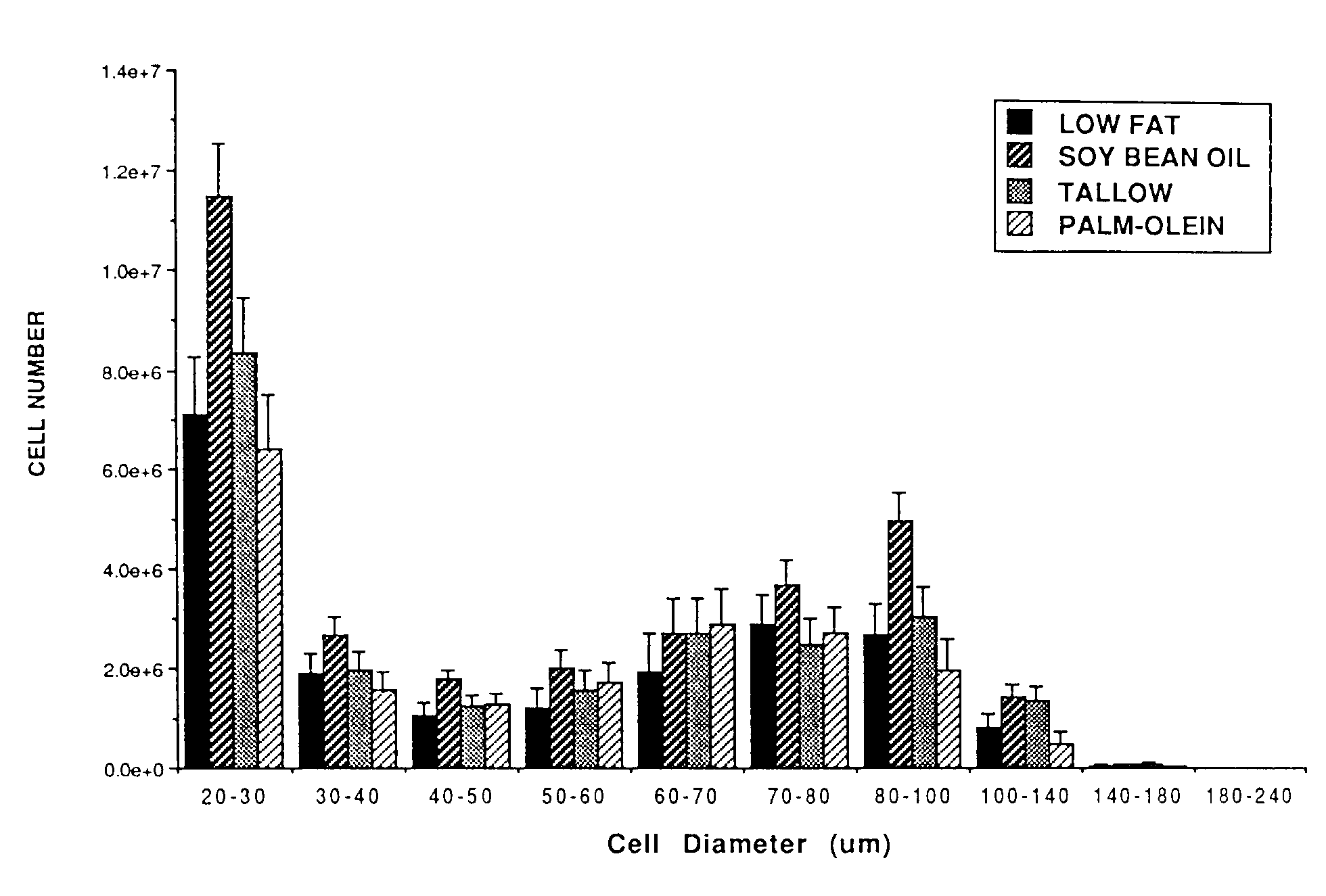
Discussion
Results of this study provide further evidence
that the type of fat fed in a high fat diet can influence lipid accretion,
adipose tissue cellularity and the development of obesity. Notably,
we observed increases in body lipid content and fat pad weight and
alterations in adipose tissue cellularity in rats fed for twelve weeks
diets containing high levels of either soybean oil or tallow. These
alterations were not observed with long term feeding of high palm-olein
diets. Rats fed high levels of palm-olein for twelve weeks maintained
a body composition, fat pad weights and adipose tissue cellularity
characteristics generally similar to the low fat fed rats.
The apparent "obesity resistance" in the
high palm-olein fed rats was unexpected and did not appear to be due
to any readily discernible effect of the diet on digestibility, energy
intake or energy expenditure. Digestibility determinations conducted
during the feeding trial indicated a higher digestibilty for the low
fat diet as compared to the three high fat groups. This was primarily
due to the increased fiber (Cellufil) in the high fat diets. Digestibilty
values for the palm-olein diet, although somewhat lower than those
previously reported44,45, were comparable
to those of the other two high fat diets. Estimated total digestible
energy intake, although somewhat higher in the tallow fed rats, was
similar in the soybean oil and palm-olein fed groups which differed
considerably in body lipid content. Likewise, daily energy expenditure
as determined by indirect calorimetry was similar in the three high
fat fed groups. Furthermore, total weight gain during the twelve week
feeding trial was similar for all dietary groups, only the composition
of gain was altered. The high fat soybean oil and tallow fed rats
apparently partitioned more energy to fat storage than did the low
fat and high fat palm-olein fed rats.
Measurement of metabolic indices was not included
in this study. Therefore, it is difficult to predict the factors responsible
for the presumed differences in nutrient partitioning between the
various high fat groups. A potential mechanism associated with this
effect could be a reduced rate of triglyceride clearance, secondary
to reduced lipoprotein lipase activity. Several studies have indicated
lower rates of post heparin plasma lipoprotein lipase activity39,46-48
and an accompanying reduction in triglyceride
clearance39,46,47 in animals fed saturated fat
as compared to unsaturated fat diets. Although these studies did not
include direct comparisons of the specific dietary fats used in the
present study, palm oil was included as the source of fat in two of
the previous experiments. Thus, Groot et al46 observed
that palm oil triglycerides were catabolized slower than sunflower
seed oil triglycerides; while Lai and Ney39 found that
postheparin plasma lipoprotein lipase activity was generally greater
and plasma triglyceride concentrations lower with ingestion of corn
oil as compared to palm oil. It is unknown whether palm-olein, a modified,
less saturated form of palm oil, would behave similar to native palm
oil in this regard. In addition, these studies of the influence of
dietary fat on lipoprotein lipase activity measured only postheparin
plasma lipoprotein lipase activity and not enzyme activity in specific
peripheral tissues. Alterations in peripheral tissue enzyme activity
would more likely be associated with differences in nutrient partitioning.
Furthermore, as body weight and fat pad weights were lowest in the
dietary group with the intermediate level of fat saturation, it may
seem implausible that the apparent differences in lipid deposition
could be explained primarily by alterations in triglyceride clearance
secondary to dietary fat-type induced alterations in lipoprotein lipase
activity. However, recent evidence indicates that the metabolic effects
of dietary triglycerides depend not only on their fatty acid composition
but also on the stereochemical configuration of the specific fatty
acids on the triglyceride molecule49,50. In order to ascertain
whether the differences in lipid deposition associated with the various
high fat diets may indeed be accounted for by diet-induced alterations
in lipoprotein lipase activity, the activity of this enzyme is being
measured in selected adipose tissue depots in our on-going studies.
The increased lipid deposition observed with the high
fat soybean oil and tallow diets in the present study was somewhat
modest (25-35% increase in percent body fat and 27-57% increase in
weight of specific fat pads) in comparison to the level of obesity
(200-250% increase in carcass lipid) reported in several previous
high fat feeding experiments10-13,51. These studies were
generally conducted for a longer period of time and/or used high fat
diets that were prepared by adding lard, vegetable oil or other fat
to the standard chow diet. Such diets induced a greater degree of
hyperphagia10,13 than was observed with the semi-purified
diets fed in the present study. It is interesting, however, that despite
the lack of effect of diet on body weight and moderate influence of
dietary fat type on carcass lipid, significant increases in adipose
tissue cellularity were observed after only twelve weeks of feeding
the high fat soybean oil and tallow diets. This alteration is important
in that changes in adipose tissue cellularity are believed to influence
the reversibility of the obese state10,12,13. Previous
studies have observed that the changes in fat cell number induced
by high fat feeding were permanent, as substitution of the high fat
diets with low fat diets lead to reductions in body weight and fat
cell size but not in fat cell number12,13. Thus, the increases
in the number of cells in the inguinal fat pad of the soybean oil
fed rats and in the epididymal fat pad of both the soybean oil and
tallow fed rats reported here would be predictive of permanent alterations
in adipose tissue cellularity in these models. This would lead to
a permanent increase in the capacity for lipid storage and adipose
tissue expansion in these animals and could thereby allow for the
induction of a greater degree of obesity with longer-term feeding
of the high fat diets.
Finally, it was noted that the increases in cellularity
observed with the high fat soybean oil and tallow diets were associated
with increases in the number of cells in both the smallest (20-30
mm) and in several larger (80-100 and 100-140 mm) size ranges. This observation
is consistent with the "critical fat cell size" hypothesis,
proposed originally by Faust et al12, which
suggests that once the peak capacity for lipid storage or "critical
fat cell size" is reached in high fat fed animals the proliferation
of adipocyte precursor cells is stimulated, triggering an increase
in fat cell number. Though generally accepted, this hypothesis has
not been thoroughly tested over the course of obesity development
and the proposed "critical" fat cell size has never been
defined. However, recent studies in our laboratory have noted increases
in the total number of fat cells, associated with a bi-modal increase
in number of both small and large cells, in genetic (B.
Marques, unpublished data) and diet-induced16 rat models
of obesity. These changes in adipose tissue cellularity were associated
with the appearance of a locally produced factor(s) capable of stimulating
adipose cell proliferation16,52. It is unknown, however,
whether or not dietary fat type could influence the endogenous production
of such local adipogenic factors and thereby contribute to the alterations
in lipid accretion observed with the various types of dietary fat
in the present study. This possibility remains the object of our on-going
investigations.
Acknowledgments
The authors express sincere appreciation to Barbra
Rose for conducting the calorimetry studies, to Amanda Latimer for
technical assistance and to Dr. Ronald Eitenmiller, University of
Georgia, Dept. of Food Science for assistance in obtaining and working
with the palm-olein. This research was supported in part by an Undergraduate
Research Grant from the University of Georgia, College of Family and
Consumer Sciences to Mee Yow Loh.
References
- National Research Council (NRC). In: Diet and Health:
Implications for Reducing Chronic Disease Risk. National Acad Press.
Washington, DC, 1989.
- Hubbard VS. Prevention of obesity: Populations
at risk, etiologic factors and intervention strategies-introduction.
Obesity Research. 1995; 3: 75S-76S.
- National Task Force on the Prevention and Treatment
of Obesity. Towards prevention of obesity. Obesity Research. 1994;
2: 571-584.
- Seidell JC. Obesity in Europe. Obesity Research.
1995; 3: 89S-94S.
- Popkin BM, Paeratakul S, Zhai F, Ge K. A review
of dietary and environmental correlates of obesity with emphasis
on developing countries. Obesity Research. 1995; 3: 145S-154S.
- Hodge AM, Dowse GK, Zimmet PZ, Collins VR. Prevalence
and secular trends in obesity in Pacific and Indian Ocean island
populations. Obesity Research. 1995; 3: 77S-88S.
- Sawaya AL, Dallal G, Solymos G, deSousa MH, Ventura
ML, Roberts SB, Sigulem DM. Obesity and malnutrition in a shantytown
population in the city of Sao Paulo, Brazil. Obesity Research. 1995;
3: 107S-116S.
- Andersson LB. Genes and obesity. Annals of Med.
1996; 28: 5-7.
- Ramirez I, Friedman MI. Dietary hyperphagia in
rats: role of fat, carbohydrate and energy content. Physiol Behav.
1990; 47:1157-1163.
- Bartness TJ, Polk DR, McGriff WR, Youngstrom TG,
DiGirolamo M. Reversal of high fat diet-induced obesity in female
rats. Am J Physiol.1992;263:R790-797.
- Hill JO, Fried SK, DiGirolamo M. Effects of a high
fat diet on energy intake and expenditure in rats. Life Sci. 1983;
33:141-149.
- Faust IM, Johnson PR, Stern JS, Hirsch J. Diet-induced
adipocyte number in-crease in adult rats: a new model of obesity.
Am J Physiol. 1978;235:E279-E286.
- Hill JO, Dorton J, Sykes MN, DiGirolamo M. Reversal
of dietary obesity is influenced by its duration and severity. Int
J Obesity 1989; 13:711-722.
- Kirtland J, Gurr MI, Widdowson EM. Body lipids
of guinea pigs exposed to different dietary fats from mid-gestation
to 3 months of age. I. the cellularity of adipose tissue. Nutr Metab.
1976; 20: 338-350.
- Klyde BJ, Hirsch J. Increased cellular proliferation
in adipose tissue of adult rats fed a high fat diet. J. Lipid Res.
1979; 20:705-715.
- Marques, BG, Hausman DB, Grossman BM, Martin RJ.
Diet, adipose cellularity and local growth factors in obesity. FASEB
J. 1996; 9:A188.
- van Amelsvoort JMM, van der Beek A, Stam JJ. Effects
of the type of dietary fatty acid on the insulin receptor function
in rat epididymal fat cells. Ann Nutr Metab. 1986; 30: 273-280.
- Clandinin MT, Foot M, Robson L. Plasma membrane:
can its structure and func-tion be modulated by dietary fat? Comp
Biochem Physiol. 1983; 76B: 335-339.
- Neelands PJ, Clandinin MT. Diet fat influences
liver plasma-membrane lipid composition and glucagon-stimulated
adenylate cyclase activity. Biochem J 1983; 212: 573-583.
- McMurchie EJ, Patten GS, McLennan PL, Charnock
JS. The interaction of dietary fatty acid and cholesterol on catecholamine-stimulated
adenylate cyclase activity in the rat heart. Biochim Biophys Acta.
1987; 898:137-153.
- McMurchie EJ, Patten GS, McLennan PL, Charnock
JS, Nestel PJ. The influence of dietary lipid supplementation on
cardiac b-adrenergic receptor adenylate cyc-lase activity in the
marmoset monkey. Biochim Biophys Acta. 1988;937:347-358.
- Field CJ, Toyomizu M, Clandinin MT. Dietary fat
and the diabetic state alter insulin binding and the fatty acyl
composition of the adipocyte plasma membrane. Biochem J. 1988; 253:417-424.
- Yakubu F, Lin D, Peters JC, Reed GW, Hill JO. Insulin
action in rats is influenced by amount and composition of dietary
fat. Obesity Research 1993; 1: 481-488.
- Awad AB, Chattopadhyay JP. Effect of dietary saturated
fatty acids on hormone-sensitive lipolysis in rat adipocytes. J
Nutr. 1986; 116: 1088-1094.
- Awad AB, Zepp EA. Alteration of rat adipose tissue
lipolytic response to norepinephrine by dietary fatty acid manipulation.
Biochem Biophy Acta. 1979; 218: 296-307.
- Larking PW, Nye ER. The effect of dietary lipids
on lipolysis in rat adipose tissue. Br J Nutr. 1975; 33: 291-297.
- Pawar SS, Tidwell HC. Effect of prostaglandin and
dietary fats on lipolysis and esterification in rat adipose tissue
in vitro. Biochim Biophys Acta 1968; 164: 167-171.
- Shimomura Y, Tamura T, Suzuki M. Less body fat
accumulation in rats fed a saf-flower oil diet than in rats fed
a beef tallow diet. J Nutr. 1990; 120: 1291-1296.
- Pan DA, Storlein LH. Dietary lipid profile is a
determinant of tissue phospholipid fatty acid composition and rate
of weight gain in rats. J Nutr. 1993; 123: 512-519.
- Su W, Jones PJH. Dietary fatty acid composition
influences energy accretion in rats. J Nutr. 1993; 123: 2109-2114.
- Hill JO, Peters JC, Lin D, Yakubu F, Greene H,
Swift L. Lipid accumulation and body fat distribution is influenced
by type of dietary fat fed to rats. Int J Obesity 1993; 17: 223-236.
- Shillabeer G, Lau DCW. Regulation of new fat cell
formation in rats: the role of dietary fats. J Lipid Research 1994;
35:592-600.
- Chong Yoon Hin. Facts about Palm Oil. Palm Oil
Res Institute of Malaysia 1987.
- Kris-Etherton PM, Ho CY, Fosmire MA. The effect
of dietary fat saturation on plasma and hepatic lipoproteins in
the rat. J. Nutr. 1984; 114: 1675-1682.
- Sundram K, Khor HT, Ong AS, Pathmanathan R. Effect
of dietary palm oils on mammary carcinogenesis in female rats induced
by 7,12-dimethylbenz(a)-anthracene. Cancer Res. 1989; 49: 1447-11451.
- Elson CE. Tropical oils: nutritional and scientific
issues. Crit Rev Food Sci Nutr. 1992; 31: 79-102.
- Ip C, Carter CA, Ip MM. Requirement of essential
fatty acid for mammary tumorigenesis in the rat. Cancer Res. 1985;
45: 1997-2001.
- Halminski MA, Marsh JB, Harrison EH. Differential
effects of fish oil, safflower oil and palm oil on fatty acid oxidation
and glycerolipid synthesis in rat liver. J Nutr. 1991; 121: 1554-1561.
- Lai H-C, Ney DM. Corn oil, palm oil and butterfat
fractions affect postprandial lipemia and lipoprotein lipase in
meal-fed rats. J Nutr. 1994; 125: 1536-1545.
- Cartwright AL. Determination of adipose tissue
cellularity. In: Biology of the Adipocyte (Eds. Hausman and Martin),
Van Nostrand Reinhold, New York, 1987; 229-254.
- Hirsch J, Gallian E. Methods for the determination
of adipose cell size in man and animals. J Lipid Res. 1968; 9: 110-119.
- Harris RBS, Martin RJ. Specific depletion of body
fat in parabiotic partners of tube-fed obese rats. Am J Physiol.
1984; 247: R380-R386.
- SAS. SAS Users Guide: Statistics. SAS Inst
Inc Cary, NC 1985.
- Calloway DH, Kutrz GW. The absorbability of natural
and modified fats. Food Research 1956; 21: 621-629.
- Ney DM, Lai H-C, Lasekan JB, Lefevre M. Interrelationship
of plasma triglycerides and HDL size and composition in rats fed
different dietary saturated fats. J Nutr. 1991; 121: 1311-1322.
- Groot PHE, deBoer BCJ, Haddeman E, Houtsmuller
UMT, Hulsmann WC. Effect of dietary fat composition on the metabolism
of triacylglycerol-rich plasma lipoproteins in the postprandial
phase in meal-fed rats. J Lipid Research 1988; 29: 541-551.
- Bagdade JD, Hazzard WR, Carlin J: 1970. Effect
of unsaturated dietary fat on plasma lipoprotein lipase activity
in normal and hyperlipidemic states. Metabolism. 19: 1020-1024.
- Coiffier E, Paris R, Lecerf J. Effects of dietary
saturated and polyunsaturated fat on lipoprotein lipase and hepatic
triglyceride lipase activity. Comp Biochem Physiol. 1987; 88: 187-92.
- Small D. The effects of glyceride structure on
absorption and metabolism. Annu Rev Nutr. 1991; 11: 413-434.
- Renaud SC, Ruf JC, Petithory D. The positional
distribution of fatty acids in palm oil and lard influences their
biologic effects in rats. J Nutr. 1995; 125: 229-237.
- Schemmel R, Mickelsen O, Gill JL. Dietary obesity
in rats: body weight and fat accretion in seven strains of rats.
J Nutr. 1970.; 100: 1041-1048.
- Marques BG, Hausman DB, Martin RJ. Influence of
adipose tissue conditioned media from obese Zucker rats on the proliferation
of rat preadipocytes in culture. J Cell Biochem. 1994; Supplement
18A: 162.
Adipose tissue expansion and the
development of obesity: influence of dietary fat type
DB Hausman, MY Loh, WP Flatt, and RJ Martin
Asia Pacific Journal of Clinical Nutrition (1997) Volume 6, Number
1: 49-55
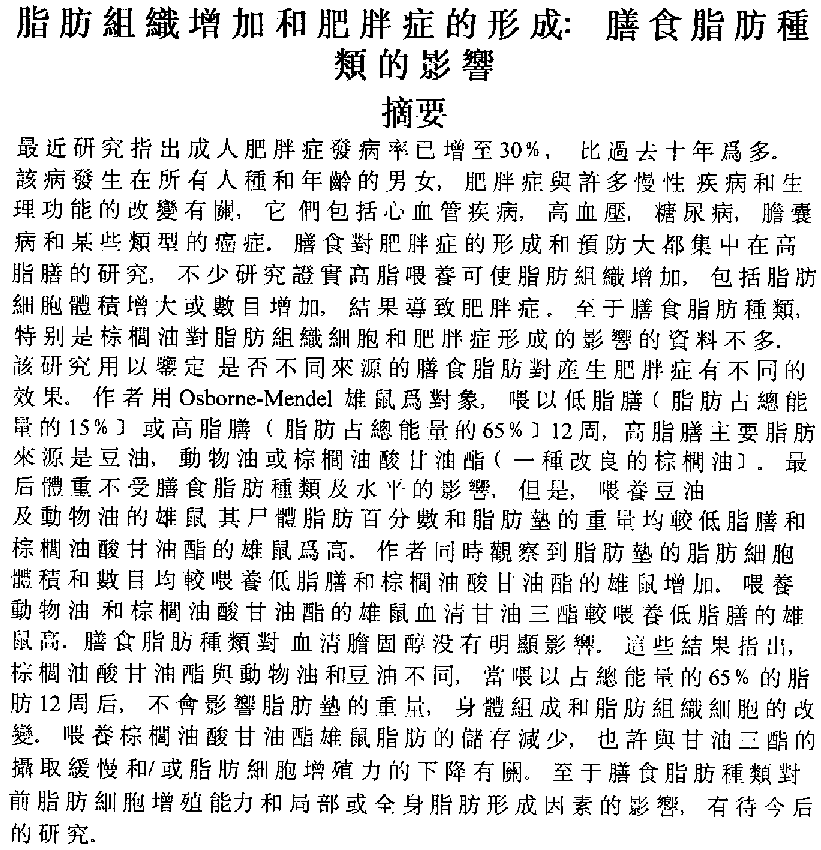

Copyright © 1997 [Asia Pacific Journal of Clinical Nutrition]. All
rights reserved.