1000
Asia Pacific J Clin Nutr (1996) 5: 59-69
Asia Pacific J Clin Nutr (1996) 5: 59-69
Review article
The
determinants of stunting: Can we regard the linear growth performance
as a continuum of fetal development?
Patrick Kolsteren
Institute of Tropical Medicine, Antwerp,
Belgium
The relationship between early post-natal growth
and the possible links with intra-uterine development is emphasised
in this review. In some Asian populations linear growth faltering
starts very early after birth and the deficit is most marked in
the first six months of life. Catch-up growth, later in life, is
possible. Children in developing countries, however, will most often
become short adults. The environment is not permissive for a catch-up
growth. A conceptual model has been constructed and divided in two
parts: intra-uterine factors and factors in the first year of life.
Only those determinants which the author considered important for
the link between fetal life and early post-natal linear growth are
analysed.
Introduction
Linear growth retardation is perhaps the most prevalent
worldwide nutritional problem. According to UNICEF1, 7.8% of the worlds
children are too thin and 42.2% are too short. South Asia is the worst
affected area where 62.5% of the children below the age of five are
too short.
The importance of short stature is to be found in
the combination of its prevalence and the human suffering it represents.
This suffering relates to the association of short stature and other
outcomes such as pregnancy risk and work capacity, but even more so
to the processes which lead to this phenomenon. Indeed short stature
can be regarded as an indicator of the existence of events which affect
linear growth and also morbidity and mortality.
The available evidence, on the determinants of stunting,
indicates that stunting is an extremely complex and multi-causal phenomenon.
The present review constructs a conceptual model which finds its legitimacy
in the classical paradigm of experimental science: first construct
a hypothesis, then derive from it a model which is the simplified
representation of reality to be tested experimentally. In the present
study, where determinants were found to be logical or where circumstantial
evidence could substantiate their place in the model they where kept
and serve as hypotheses to be tested further. The model is represented
in Figures 1-3.
Figure 1. Linear growth retardation
in the first year of life. |
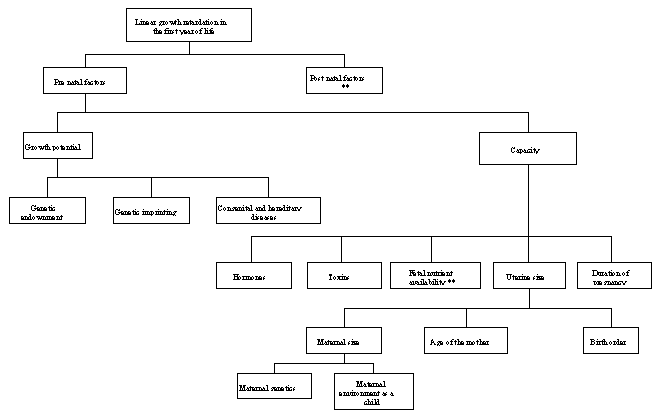 |
Figure 2. Fetal nutrient availability. |
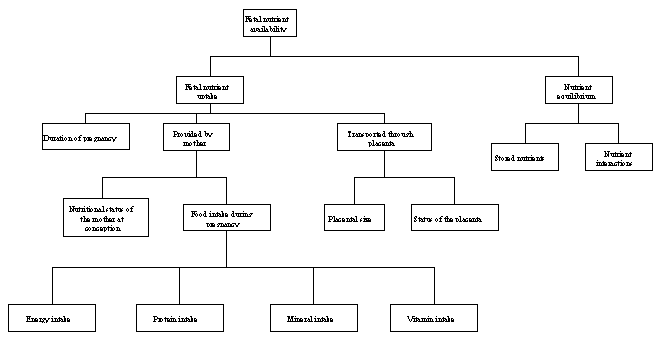 |
Figure 3. Post natal factors. |
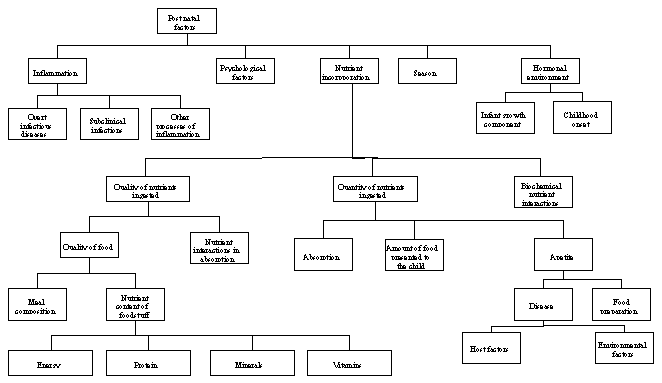 |
The main hypothesis put forward in the analysis is
that the growth of children in the first year of life could, in part,
be determined by fetal life experience.
A first evidence for this comes from a biologically-oriented
mathematical model (ICP model) where the linear growth of children
from birth to adulthood is modelled on the basis of three phases:
Infancy, Childhood and Puberty2. For all three of the phases,
a mathematical function describes the growth curves of children. These
functions are additive, in the sense that the infancy function describes
the growth of children up to the moment childhood growth starts, described
by another function which is then added to the infancy curve.
The existence and shape of the infancy component of
the ICP growth model is supported by the growth of children with growth
hormone deficiency. They show a growth pattern for supine length close
to the reference values given by the infancy function from birth up
to the start of substitution therapy at about 2 years of age3.
Infants with a late onset childhood component tend to continue growing
according to the infancy growth component until the childhood growth
phase starts4-6.
If one extrapolates values for the mean infancy function,
that is the values of attained height derived from the fitted curve
on the observed values of infants, into fetal life, one observes that
the extrapolated values are close to the mean values for cross-sectionally
measured supine length of fetuses from about mid-gestation onwards2.
Karlberg who studied different populations using his
ICP model concludes that during the period preceding the age of onset
of the childhood component, 83% of normal infants have a non-linear
decelerating growth pattern, free from seasonal variation. For the
majority of the infants in this phase growth seems very stable over
time. We can add to this the information that the hormonal control
of growth in the first months after birth is similar to the intra-uterine
one. Indeed the resemblance in hormonal control between intra-uterine
life and the first months of neonatal life are extraordinary. The
childhood component of linear growth is triggered by the onset of
growth hormone secretion.
Could it be possible that the infancy growth and fetal
growth are very intricately interwoven, and form perhaps one continuum?
How should we then interpret the observations that growth faltering
start early?
Very few longitudinal studies have actually investigated
when growth faltering starts. Cross sectional studies conclude that
it indeed starts early, around two months and possibly earlier7.
The possibility arises here that we are observing an artefact and
that growth is sub-optimal from birth onwards and therefore determined
by intra-uterine factors. This artefact might be the result of the
observations being predominantly cross-sectional. If the average z-score
of the studied population is below zero at 2 months, one decides that
this is the period when growth faltering is first observed. But as
Waterlow8 and Golden9 describe, it takes time
to become stunted. The time is inversely proportionate to the magnitude
of the growth deceleration when using z-scores. If we observe a difference
at two months in z-score this would lead us to conclude that the process
of stunting is already of a longer duration.
One supplementation stu 1000 dy from Indonesia showed
an effect on linear growth post natally when the mothers were supplemented
during pregnancy. Supplements given to pregnant women resulted in
an improved growth performance of their offspring in the first 3-6
months of life for those children whose mothers received a high energy
supplement as compared to those who received a low energy supplement10.
Although length at birth was comparable in the two groups, the high
energy group maintained a high growth velocity in the first months.
Children whose mothers had received a high energy supplement during
pregnancy were still significantly taller at the age of five years.
Length at birth was found to be associated with indicators of social
status in Karthoum, where mothers with higher incomes and levels of
education, tended to have longer children11.
Based on this we have considered that early post-natal
growth can be influenced by prenatal factors, which not only determine
birth size, but also exert their influence on linear growth in the
first months of life. Growth in the first months of life may be a
continuum with intra-uterine growth, particularly with its second
half. Nevertheless, the dichotomisation of post natal versus pre natal
factors may be kept in the model.
Birth size
Birth size has been studied extensively as a determinant
of further outcomes like morbidity, mortality and subsequent growth
and development12-22. In these studies it has mainly been
birth weight, or more precisely weight for age, which has been taken
as an indicator. The results of the studies are difficult to interpret
and often give conflicting results mainly because weight for age is
a very global indicator.
The use of weight for age as an indicator of nutritional
status is limited and a classification based on height for age and
weight for height12,23 often preferred; children are classified
as stunted, wasted, normal, or stunted and wasted. Birth weight alone
fails to diagnose intrauterine malnutrition24. Clinical
diagnosis of malnourished infants at birth better identifies those
children who later develop complications related to their malnutrition;
39% of malnourished newborns were missed by using weight as an indicator
alone25.
Another problem with considering birth weight as a
determinant is that it leads to a conceptual mix up between an indicator
and a determinant. It could be that size at birth is a proxy indicator
of a phenomenon rather than a real determinant, or that some of the
determinants of birth-size, which are multiple, also affect linear
growth whereas others not. Other literature is available on the subject
and not included here.
To meet some of the deficiencies of using birth weight
alone, a dichotomous classification of small-for-gestational age (SGA)
newborns has been proposed. Low birth-weight infants are classified
as disproportionately growth-retarded infants, who have a relatively
normal length and head circumference for their gestational age but
low weight, and proportionately intrauterine growth retarded infants
who have symmetric growth reductions in weight, length, and head circumference24,26.
This would then cover the wasted children (disproportionate) and a
group of children either stunted or stunted and wasted (proportionate
growth retarded). This classification does not cover newborns who
are stunted with a normal birth weight.
Miller and Hassanein27, revisiting the
diagnostic criteria for intra uterine growth retardation, demonstrate
the inadequacy of birth weight alone to evaluate fetal nutrition adequacy
and give examples of infants with normal birth weights and variable
high and low ponderal index (weight/ht3) but respectively
short and high nor 1000 mal stature. Most authors who propose a different
approach from birth weight alone do so conceptually as if there are
two different kinds of growth retardation; one more chronic starting
early and affecting the fetus when still in a "growth" phase,
ie; a phase of cell replication, and another when predominantly becoming
bigger and depositing fat, ie towards the end of pregnancy. The first
insult would predominantly affect linear growth, the second one predominantly
the weight of the child. Hence the dichotomisation of proportionate
and disproportionate intrauterine growth retarded infants would differentiate,
respectively, the chronically malnourished infants from the acutely
malnourished ones. Each type of intrauterine malnutrition may have
a different outcome20,22,24.
The problem with most studies is that they start by
dichotomising the infants first on a weight for age basis, to classify
them as intrauterine growth retarded, and then for the growth retarded
children as proportional and dis-proportional.
When we look at the outcomes of intrauterine growth
retarded infants (the small-for-dates) we find on the whole that they
are smaller in length at birth with smaller head circumferences. They
catch up growth, both in length and in weight during the first three
two six months16,17,19-22. Their catch-up is, however,
not complete with a deficit remaining at two years. Follow-up of the
children in one study showed that, even at the age of 19, the deficit
remained18. According to Falkner this is an oversimplification28.
Small for date infants, or small for gestational age neonates, are
a heterogenous group. Some children seem to catch up and others do
not. Most of the asymmetric SGA infants show a postnatal catch-up
and grow well. A small proportion exhibit some catch-up but, at least
by two years, do not reach the 5th centile.
Pre-term infants also show an intensive phase of catch-up
growth which is sustained longer than for the small-for-dates so that
by the age of three years they have caught up with normal controls16,19.
The growth pattern of the small-for-dates is very heterogenous with
a wide variety of growth velocities. Infants with a low ponderal index
(wasted ones) do better after birth in terms of catch-up growth than
the ones with a normal ponderal index ( those who are most probably
also short)21.
In infants who are not growth retarded, ie children
with a normal birth weight, further growth is not correlated with
their birth weight. Correlations between birth size and subsequent
growth velocities are near zero for weight and weight velocity and
length and for length velocity29. When maternal weight
is taken into account there are differences in long term weight gain.
Low birth weight babies of low weight mothers grow at a slower rate
than comparably low birth weight babies from high weight mothers30.
One likely explanation is that the subsequent growth of the neonate
reflects maternal body mass and the low weight infant born to the
high weight mother is simply returned to a genetically programmed
development course. This shifting of growth post natally has also
been proposed as an explanation by other authors31 who
followed 90 children from a well-off background from birth. The correlation
between length at birth and length at one and two years was very poor;
however, by age one year the correlation to length at age two years
is good for both girls and boys indicating that a proportion of the
children shift. The shifting has two directions: upward and downward.
Children born at the lower extremes start to cross growth percentile
lines early after birth and achieve a stable growth percentile at
the age of one year. Infants at the upper range of length follow their
percentile for three months until they start to dece 1000 lerate in
growth32.
Genetic endowment
Genetic endowment has an important influence on attained
height and therefore on the way a child will grow in the first year
of life. Habicht33 concluded that genetic potential does
influence the observed difference in attained height but that the
accumulated deficit indicates that genetics play a lesser role than
environment. This has been confirmed8,31,32,34-38.
Rona34 analysed studies of childrens
height, from a genetic view-point in two ways: one was to assess difference
in growth between ethnic groups and the other to investigate anthropometric
measurements of relatives sharing identical genes. The studies of
ethnic groups have compared the same ethnic groups with shared genetic
endowment, living in different environments and different ethnic groups
living in similar environments. These provide evidence for the much
more important influence of environment on adult stature.
Family studies which compare mono-zygotic and di-zygotic
twins find correlations of height of the order of 0.93 by the age
of three years for mono-zygotic siblings and 0.79 for dizygotic twins.
In large samples of more than 500 pairs, most studies find parent
- child correlations of 0.3-0.4 for attained height. However it is
difficult to separate common environmental influences from genetic
influence in theses studies. Adoptive parent-child correlations are
0.30, slightly lower than the 0.35 values quoted for parent-child
relations. This suggests that common environment and behaviours play
an important role.
We can conclude that genetics play an important role
but have minor potential to influence growth compared with the environment34.
Genetic imprinting
Genetic imprinting is an epigenomic phenomenon, which
switches genes off or on during the early stages of development. These
switches can persist in childhood and adulthood. Golden9
notes that the smaller newborn of a monozygotic twin pair will end
up as an adult of smaller size. Rats who, during their development
receive a restricted diet, will, once they return to a normal diet,
have larger offspring, it takes three generations for them to attain
the size of the control group.
Mice on a zinc deficient diet demonstrate this "inheritable
effect". The pups of mice, fed a zinc deficient diet during pregnancy
become immuno-deficient. The offspring of these immuno-deficient mice
are partially immuno-deficient. It takes three generations to restore
normality.
Variation in imprinting by specific epigenomic modification,
and contributed by poor nutrition, provides an explanation for the
above observations. Since oogenesis occurs in fetal life, the nutritional
plane of the grandparents may influence the grandchild and be as important
as the nutrition of the mother. If the meiotic and early in utero
environment changes the pattern of epigenomic modification to alter
the potential for future somatic development over several generations,
it would provide an explanation for the close association between
height potential and familial height in societies were there are no
racial differences in height. It also provides an explanation for
the gradual secular trend in height which clearly transcends the genes
in the germ line and yet is familial.
In The Netherlands a similar phenomenon was observed
in relation to the Dutch winter hunger. Not only were the children
born to mothers who suffered famine lighter at birth, but so also
was the second generation of offspring. This "carry over"
effect was manifest in the offspring of women conceived during the
famine. The timing of the intra uter 1000 ine exposure to undernutrition
appears in this study to be crucial39.
Congenital and hereditary diseases
Short stature may typify congenital or hereditary
disease. Although medically important and as indirect evidence of
genetic involvement in stature, these diseases are less important
for the study of stunting at a population level. Analysis and enumeration
of congenital and hereditary disease in the aetiology of stunting
is not pursued further in this review.
Fetal nutrient availability
This determinant of stunting has been dichotomised
as shown in Figure 2.
Food intake of the mother affects intrauterine development
and growth, most frequently measured by birth weight. Available information
comes from three distinct types of studies:
- Historical ones where experimental starvation
designs were imitated by natural calamities, such as the Dutch winter
hunger of 1944-45 and the siege of Leningrad.
- Epidemiological studies, involving large
samples of the population, of the relationship between fetal growth
and development with diet during pregnancy, weight gain during pregnancy,
BMI pre-pregnancy and post-pregnancy and socio-economic status40-44.
- Clinical supplementation studies in both
developing countries and developed countries40,45-58.
To take these in turn
- During the Dutch winter-hunger, a famine hit the
west of Holland and lasted 27 weeks. Dietary restriction lasted
for six months and was the result of a strict rationing of food
to 750 Kcal and 28 g protein per day. After some delay a sharp decline
in maternal weight followed the decline in energy intake. Those,
exposed in the third trimester suffered an average loss in postpartum
weight of 5.12kg. Birth weight followed in turn with a decline of
320g from an average birth weight of 3327g, in the cohort with the
same third trimester exposure. In reverse, when normal rations were
restored upon liberation of Holland, the same sequence held for
women in the third trimester; recovery of 5.7kg in postpartum maternal
weight was followed in birth weight of 380g to a mean of 3387g.
Adverse effects on birth weight were not noted when the daily energy
intake remained above 1500 Kcal.
- Epidemiological studies use mostly maternal weight
gain, maternal body mass index (wt/ht2), or postpartum
maternal weight as indicators of maternal food intake during pregnancy.
Maternal weight correlates with birth weight; height also correlates
with birth weight but its effect is in some studies accounted for
by the concomitant increase in weight44.
- Supplementation studies can be divided into three
categories: those where there has been a positive effect on birth
weight, no effect or effect only in certain circumstances. Some
examples follow:
- At Sorrento, Birmingham47,48,
supplementation enhanced fetal growth in mothers at nutritional
risk, but had no effect, or possibly adverse effects, in the
adequately nourished.
- Gambian findings52 have similarities.
Fetal growth was enhanced compared with historical controls
when 1000 mothers received a supplement during the rainy season,
when food was limited, and they had to work in the fields. However,
when future mothers received a supplemented during the dry season,
the more affluent season, growth was not enhanced. Indeed, it
was even slightly below the controls.
- In Indonesia an increase in birth weight
was observed in the mothers who received a high energy supplement.
In this particular group, body mass index of women in the community
was marginally low, indicating chronic energy deficiency.
- In Chile55, two groups of
future mothers received supplements during pregnancy. One was
a milk powder supplement, and the other, a fortified milk-based
supplement. This latter group had vitamin and mineral intakes
which were considerably higher for vitamins A, C, D, E, niacin,
pyridoxine, folic acid, iron, magnesium, zinc, copper and iodine.
In this study there was a large increase in birth weight in
the supplemented groups. Compared to other studies, where increases
are noted of 30-70g in birth weight, this study found increases
of 258g and 335g. The larger increase was noted in the mothers
who received the vitamin and mineral supplement. Not only was
the order of magnitude of the birth weight increase in this
study remarkable, but also
- studies describing similar increases in birth
weight are those where mothers are nutritionally worse off
48 or in a period of very low food intake52,
whereas in this particular study the average weight for height
percentage was 90% and
- the larger increase occurred in the group receiving
the mineral and vitamin supplement.
The findings may explain the small increases in birth
weight after energy and protein supplementation, in that other factors
such as minerals and vitamins limit the increase in birth weight.
Evidence for an effect of mineral supplementation itself comes from
a study59 where women at risk of delivering a small-for-dates
baby were assigned to a zinc supplement or a placebo group. The zinc
supplemented group required less medical intervention in terms of
induction of labour and Caesarean section for fetal distress. Birth
weight was higher in the supplemented group (3060g vs 2780g), but
the difference was not significant, probably due to the small numbers
in the supplemented (n=13) and control (n=16) group. Figures on length
at birth are not provided, but the ponderal index which was smaller
in the supplemented group, 0.02 vs 0.23, combined with the higher
birth weight lets us suppose that the newborns in the supplemented
group were longer.
As mentioned previously, the most frequently used
indicator of birth size, is weight. Very little information is available
on length at birth and the subsequent growth of newborns, thus, in
the following analysis birth weight and factors determining it, or
associated with it are considered, as a proxy to understand what could
determine length and subsequent growth.
These studies lea 1000 d to the following conclusions:
dietary restriction causes a decrease in birth weight while supplementation
studies show less than the desired effect; on average the birth weight
increases by 50g. High density protein supplements are consistently
associated with decreased mean birth weight, as concluded by Rush60.
Studies of the determinants of birth weight, although
they find different strengths of association or correlation, or are
in conflict have at least the following in common:
- they provide little information on cause or underlying
mechanisms. Maternal stature, birth order, age of the mother, parity
are all found to be correlated with birth weight, but provide little
information on the possible explanatory mechanisms. Susser, analysing
supplementation studies where pregnant women were given energy/protein,
found conflict in putative causal links between energy intake, or
mothers weight and birth weight53.
- multiple regression analyses reveal a large proportion
of the observed variance remains unexplained. In an observational
study in Sorrento, Birmingham, 78% of the variance was unexplained;
comparison of two groups of women ten years apart improved the results,
but still left 47% unexplained. A similar study in Kenya, found
that weight of the mother at three months pregnancy, her height,
her weight gain during pregnancy, sex of the offspring, parity and
skinfold thickness during pregnancy could only explain 30% of the
variance in birth weight40.
Micronutrients
The effects of minerals and vitamins on linear growth
have mainly been studied in infants and children. Very little is available
on the effect of vitamin and mineral status during pregnancy and linear
growth in the first year of life. (The question is which micronutrient,
if there is need of an effect, can affect linear growth?)
Minerals
The following minerals have been proposed as necessary
for optimal linear growth: calcium, phosphorous, sulphate, magnesium,
iron, iodine, zinc, copper. The elements reviewed here are zinc, iron,
copper and iodine.
Zinc
Zinc deficiency in children depresses growth, appetite,
skeletal maturation and gonadal development, which can be reserved
with Zn treatment. Zinc deficiency is associated with metabolic disturbances
of a wide range of hormones, cytokines, and enzymes involved in growth
and bone development. Zn deficiency affects the immune system, the
structure of the skin and intestinal mucosa, wound healing and dark
adaptation61. Children with severe malnutrition show clinical
signs and immunological deficits which are correctable by zinc62.
How zinc affects bone growth and maturation is not clear.
There have been a number of observations which suggest
that Zn deprivation may be implicated in human growth retardation.
Adolescent nutritional dwarfism in Middle-Eastern countries, characterised
by poor growth and delayed sexual maturity, has been related to Zn
deficiency in association with deficiencies of other nutrients. In
addition, poor Zn status, as suggested by low Zn levels in blood or
hair, has been described in growth retarded Chinese, Mexican, Thai
and Papua New Guinean children61,63.
Supplementation studies show more mixed results. Over-all
boys respond more or only to a zinc supplement. Comparison of the
different studies is difficult due to the heterogeneity of subjects,
dosages and duration of the supplements given. Despite this, the ac
1000 cumulating evidence suggests that Zn supplementation can increase
the height and weight gains of certain groups, particularly infants
and adolescent boys, in both developing and developed countries61.
The results of zinc supplementation are more dramatic
for children, male and female, recovering from severe malnutrition.
In Jamaica, children who received a zinc supplement increase their
lean body mass and total body water at the expense of adipose tissue64.
Marasmic children in Chile responded to zinc supplementation, given
for 90 days, by increasing their weight-for-length but not their length-for-age65.
Schlessinger et al observed that a zinc-fortified formula improved
linear growth of Chilean infants (average age 7 months) recovering
from marasmus, compared to a non-fortified formula containing standard
amounts of zinc (and other nutrients). The difference was not much
over a period of 105 days, but the supplemented male infants had an
earlier growth spurt. Immune response was also better in the supplemented
group, although there was no effect on the number or duration of infectious
episodes, suggesting that the beneficial effect on growth was not
explained by lower morbidity.
How zinc affects linear growth is not clear. Zinc
is a potent appetite regulator. It is also necessary for the mobilisation
of vitamin A, affecting the synthesis of apo-retinol, and its metabolism
at the periphery where it affects retinal dehydrogenase activity,
decreasing the conversion of retinol to retinal. Zinc is also an essential
element in protein synthesis, as part of DNA and RNA performance.
Iron
Evidence of the effect of Iron on linear growth is
rather scant and the results of the few supplementation studies either
do not report linear growth effects or give conflicting results63.
These mixed results might be explained in part by the difficulty of
detecting significant growth differences in the relatively short periods
during which iron supplements were given. Most probably linear growth
only responds to iron treatment if the child is initially anaemic.
Two studies from Indonesia66,67 confirm this effect of
supplementation in anaemic children on linear growth. In both studies,
anaemic children were thinner and shorter before the supplementation
and they considerably improved their weight and height after supplementation.
Whether this effect is directly related to iron or mediated by another
mechanism is not clear. Anaemic adults increase their food intake
after treatment, but, in one study where food intake was measured,
no increase in food intake was noted. Less morbid episodes could be
an explanation. Treated children showed significantly less morbid
episodes as measured by fever, respiratory infections and diarrhoea67.
In Bangladesh, children with low height-for-age indices
showed lower levels of haemoglobin, (Hb), serum copper and serum vitamin
A levels68.
Iron plays a role in the maintenance of a normal immunity
and myeloperoxidase activity of the lymphocytes is decreased in anaemic
subjects. The relationship of iron to morbidity could be explained
by a reversal of depressed immunity in these studies .
Iron also has roles as a co-enzyme. Thus it is involved
in a wide range of cellular functions from the cellular respiratory
chain and ATP generation, to amino acid metabolism and hydroxylation
of steroids. Iron supplementation in anaemic and iron deficient non-anaemic
female workers has a beneficial effect on energy expenditure and work
activity69. Supplemented workers have lower heart rate
and total energy expenditure. The change in production efficiency
after treatment is significantly correlated with the change in Hb
values, but not with the change in h 1000 eart rate at work. It is
concluded that the improvement in production efficiency is not explained
directly by the decrease in mean heart rate at work. A possible explanation
is that the specific activities of iron containing enzymes in the
mitochondrial electron transport system and related muscular efficiency
increase with improved iron status. A direct effect of iron through
cellular metabolism is likely.
Information of these kinds on the effects of supplementation
in iron deficient, as opposed to anaemic children is not available.
Copper
Children recovering from severe malnutrition have
low copper stores and benefit from copper supplementation especially
if on high energy, low copper diets39. Supplementing copper
was found to have a beneficial effect on weight gain and weight-for-length
in 11 children recovering from malnutrition. Only those children with
initially low serum copper and ceruloplasmin were found to benefit.
Energy intake was also higher after supplementation. Length increase
was greater, but not significantly so, perhaps because the period
of study was short and the number of subjects small. In Zaire, children
who failed to respond to treatment for malnutrition where found to
increase their weight and length growth after having received a copper
supplement70.
Iodine
Iodine deficiency, when severe, causes stunting. It
is a classical sign of the severe deficiency state. Marginal deficiency
is also associated with short stature71. It is even possible
that iodine deficiency during fetal life has a persistent effect on
later growth. In a region of Ecuador with severe iodine deficiency,
interventions with iodinated oil failed to change childrens
growth. On the other hand, if women are given iodinated oil prior
to conception, the birth weight of their infants is increased63.
Vitamin A
Vitamin A has been known to be required for growth
since its discovery in 191372. Deceleration in weight gain
is one of the earliest, most predictable events after acute withdrawal
of vitamin A from the diet of young animals, attributable to losses
in efficiency in food, and specifically, protein utilisation. Prolonged
deficiency exhausts circulating and hepatic levels of vitamin A causing
cessation of growth and subsequent weight loss, accompanied by reductions
in body fat and deranged protein metabolism. Resupplementation with
vitamin A restores normal growth73.
The relationship between vitamin A and linear growth
has been demonstrated in several cross-sectional and longitudinal
studies. Children with clinical signs of vitamin A deficiency are,
in most studies, found to be more stunted than children without signs74-79.
There is also a relationship between vitamin A status and wasting.
In Malawi, however, no association was found between vitamin A status
and either stunting or wasting80.
In a longitudinal prospective study in Indonesia,
4000 children were followed for a period of three months81.
Children with signs of xerophthalmia were found to grow less in weight
and height, which extrapolated over one year would amount to 0.2-1.7cm
less growth in length. After supplementation, children showed a catch
up in weight but not in length. Girls were more affected than boys
which, according to the authors, could be explained by girls having
more severe signs of xerophthalmia. Another supplementation study
in Indonesia73 found vitamin A supplementation to improve
child growth, but only in males and only in weight. Since the supplements
only raised retinol levels for 2-3 months before returning to baseline
values the authors proposed that the suppleme 1000 nt raised the retinol
values insufficiently to sustain a catch-up growth in length. This
might explain why fortification of monosodium glutamate with vitamin
A showed a strong and consistent advantage in linear growth among
programme children at every age82,83. Another possible
explanation, not researched by these studies, is the possible role
other micronutrient deficiencies may have on the effect of vitamin
A supplementation.
Interactions of micronutrients
Failure to study the effect of iron and vitamin A
on growth is partly related to the difficulty of isolating observations
to one nutrient, because a variety of nutrients interact to carry
out biological functions.
Hodges et al reviewed the conclusions of eight studies
by the US Interdepartmental Committee for National Defence on non-pregnant
and non-lactating women in developing countries where iron intake
was more than 14mg per day. There was no relation between haemoglobin
and iron intake, but a strong correlation between serum vitamin A
and haemoglobin. A positive correlation between retinol in serum and
haemoglobin in the blood of children in Ethiopia and in pregnant women
in Indonesia has been demonstrated84.
Iron deficiency is more easily corrected when there
is no vitamin A deficiency. Studies have demonstrated a higher efficiency
of the treatment of anaemia when vitamin A is added to the treatment,
even in the absence of clinical vitamin A deficiency84-86.
An explanation is not available for the observed relationship from
human studies. Prolonged vitamin A deficiency in animals negatively
affects haematopoiesis with a gradual replacement of the bone marrow
by fibrous and fat tissue. The iron absorption increases in vitamin
A deficient animals and at the same time haemosiderosis of the spleen
and the liver can be demonstrated. The phenomena reverse after vitamin
A supplementation86.
In the late 1970s, several workers tried to elucidate
the relationships between vitamin A and iron. In an experiment involving
a vitamin A deficient group of otherwise healthy volunteers, an association
between iron metabolism and hypovitaminosis A was established. The
experimental group developed anaemia refractory to medicinal iron
but responsive to vitamin A. In Central America vitamin A fortification
of sugar without an increase of iron in the diet had a positive impact
on iron metabolism87. The authors observed that after six
months of a vitamin A fortification, indices of iron status improved.
Because the levels of total iron binding capacity (TIBC) increased
and the levels of ferritin decreased, it seems that, after fortification,
there was an increase in the availability of serum iron by depletion
of the iron stores for haematopoiesis. After two years of vitamin
A fortification an overall significant improvement of all the iron
nutritional indices was observed; serum iron, percent saturation,
and ferritin had increased significantly and TIBC had decreased. A
plausible explanation for this phenomenon is that vitamin A enhances
both haematopoiesis and the availability of serum iron by depleting
the iron stores. This may trigger the improvement of dietary iron
absorption and as a consequence might lead, after two years of fortification,
to elevated levels of ferritin and a decrease of TIBC levels as may
be expected in normal iron metabolism87. These findings
were confirmed first in a cross-sectional study88 and later
in a supplementation study in Thailand89 where a single
dose of vitamin A was found to improve iron status. The difference
in serum retinol and serum retinol binding protein which increased
between the control and the supplemented group where not significant
anymore four months after intervention. These findings support the
thesis of studies in 1000 Latin America where a single dose supplement
did not affect linear growth but fortification, which provides vitamin
A for longer periods, did.
The above mentioned studies support the hypothesis
that the interaction between iron and vitamin A has three modes of
action: 1) lack of vitamin A immobilises iron stored in the reticuloendothelial
system; 2) the initial depletion of iron stores, and consequently
increased utilisation after vitamin A supplementation, triggers the
dietary absorption of iron and leads to the observed higher levels
of ferritin after four months; 3) the association between vitamin
A stores and infections leads to a decreased morbidity in children
who receive a supplement, and this in turn positively affects iron
utilisation. Zinc is also known to interact with vitamin A. Zinc deficiency
decreases levels of serum albumin, pre-albumin and transferrin. Pre
albumin levels increase rapidly with a zinc supplementation of 10-15
days90. This effect is probably mediated through the effect
of zinc on protein synthesis, most noticeable in the effect of zinc
deficiency on the synthesis of retinol binding protein91.
Here zinc deficiency is found to be necessary for normal mobilisation
of vitamin A from the liver and maintenance of normal serum levels.
A study from India involving malnourished children supports this concept.
These children showed a significant increase of plasma Vitamin A and
retinol binding protein in response to zinc supplementation. In a
study in Thailand, no adverse effect on retinol binding protein or
serum retinol was noted when zinc was added to vitamin A supplementation
as compared to children who received vitamin A alone92.
In this study, however, it was not clear whether the children were
significantly zinc deficient or to what degree.
Gestational age
This is discussed in the birth size paragraph.
Uterine size
As with other determinants of stunting, the influence
of the fetal environment on birth outcome has been studied predominantly
by using birth weight as an outcome. Some studies have, however, demonstrated
that the fetal environment does have an effect on length. A classical
experiment addressing this question is the one of Walton and Hammond93
in which offspring of matings between Shire and Shetland ponies were
studied. There is approximately a fourfold difference in weight between
the Shire and Shetland mares. The reciprocal crosses, achieved by
artificial insemination provided embryos of substantial different
genotype in uteri of different sizes. The hybrid foals born to Shetland
mares were comparable in size to pure-bred Shetland while foals born
to Shire mothers were considerably larger, approaching but not equalling
the weight of pure bred Shires. Both types of foals had normal proportions.
Similar results have been obtained in cattle and in sheep94.
Roberts35 in a synthetic article cites
an example where 38% of the variance in individual birth-weights among
surviving infants can be attributed to heredity. However this 38%,
is made up of only 16% from fetal heredity factors other than sex,
2% from fetal sex, and 20% from maternal hereditary constitution.
The greater part of the variance, the remaining 62%, was attributable
to environmental causes, of which 18% derived from the mothers
general health and nutrition, 6% from her health during each particular
pregnancy, 7% from her parity, and 1% from her age, the remaining
30% being attributable to unknown intra-uterine influences. In the
same article mention is made of a study of Morton who analysed massive
data from Japan. Account was taken of the effects of maternal age,
parity, the sex of each child, and the number of pregnancies intervening
between each pair of siblings. At birth, like-sexe 1000 d twins, with
a high degree of common heredity were not more alike than full siblings
or even half-siblings from the same mother. By contrast, the half-siblings
with the same father were notably unalike. The effect of consanguinity
was negligible, indicating no detectable effect of recessive genes.
The mothers phenotype seems to be a principle determinant of the size
of the newborn child.
Similar studies have been done by Tanner, who found
that the correlation between length at birth and adult height is very
low, about 0.3. By six months it rises to 0.5 and by one year to 0.7;
by two years a stable prepubertal level of nearly 0.8 is reached95.
In the first part of fetal development, the weight
gain of the fetus merely reflects cell growth. A period of linear
peak growth velocity can be assumed later on, but the gestational
age at which this occurs is still somewhat uncertain with estimates
for 16 weeks of gestation28. After this, linear growth
velocity decreases and continues to do so well into the postnatal
period28. According to the same author, fetal growth slows
down at 34-36 weeks owing to space constraints. Twins slow down considerably
earlier when their combined weight is approaching that of a 36-week
singleton fetus.
A problem in the construction of a causal model is
the question of a primary operational focus. Should we put height
of the mother as a determinant of uterine size? Do we have any indication
that taller women have more space to accommodate a bigger uterus and
so allow for taller children. Is that the mechanisms by which maternal
height effects it influence?
We reflect a similarities on birth order. Why are
first born children lighter? Does the uterus have to go through an
adaptation process by which it is smaller in the first pregnancy and
becomes bigger afterwards? Is this a stretching phenomenon?
How does the age of the mother affect the size of
the offspring? Younger women are not full grown and have lesser space
for the uterus. Is this the explanation? Age of the mother may determine
uterine size and fetal outcome.
Placental size
Falkner28 quotes the Louisville Longitudinal
Study of Twins which provides evidence of a strong correlation between
placental weight and birth weight. In monozygous twins, who are phenotypically
similar, differences in birth weight and in postnatal growth may be
determined by placental weight. The concentrations of several biochemical
and nutritional substances are the same in the placental segments
belonging to each twin, but, because of the difference in placental
weights, the absolute amounts are different.
The hormonal environment
Post natal growth depends on four main hormonal regulating
mechanisms: a) Growth hormone via somatomedin, b) thyroid hormone,
c) cortisol and d) sex hormones during adolescence .
Insulin has a more permissive role in post natal growth96.
The fetus in contrast depends less on growth hormone and thyroid hormone
for its growth and more on insulin and tissue growth factors.
Maternal growth hormone does not cross the placental
barrier, making the fetus entirely dependant on its own hormone homeostasis
for growth.
The role of growth hormone in fetal growth has been
studied in different species by depriving the fetus of its pituitary
gland in utero. Normal fetal growth is possible when growth hormone
is lacking or is markedly reduced. GH-dependant growth is not observed
until well after birth; in humans at six months. The hypothesis that
growth hormone is not an important determinant of fetal or neonatal
growth has been advanced by Karlberg 1000 2,5 who based
his ICP model for growth on this concept. Growth hormone dependence
becomes noticeable with the onset of the childhood component of growth
in this model. Intrauterine hormonal homeostasis seems to go beyond
intrauterine life.
Thyroid hormones are also not transferred from the
mother to the fetus and the fetus is dependant on its own thyroid
gland for thyroid hormone production. Human newborns suffering from
congenital hypothyroidism, or radiothyroidectomised in utero by radioactive
iodine given inadvisedly to the mother, have a normal size and weight
at birth96. Thus thyroid hormones appear to have little
effect on fetal growth in most species, but they are essential for
normal neural and osseous maturation97.
Insulin
The concept that insulin might be an important hormone
for fetal growth arose primarily from clinical observations with insulin
excess or insulin deficiency. The infants of diabetic mothers are
larger (on average 500g) and somewhat longer (on average 1.5cm) than
control infants98, though most of the overweight is due
to lipid deposition. Since the excessive growth becomes obvious after
the 28th week99, at a time where the fetal pancreas becomes
sensitive to glucose100, it has been suggested that maternal
hyper-glycaemia is attended by fetal hyperglycaemia which, in turn,
stimulates insulin secretion by the fetal pancreas and induces macrosomia.
In contrast, newborns with pancreatic agenesis have profound intrauterine
growth retardation associated with deficient adipose tissue and a
decease in muscle mass101. Infants with transient neonatal
diabetes also have a defect in insulin secretion which is associated
with a low birth weight102. These infants have reduced
adipose tissue and reduced muscle mass which undergoes rapid development
with postnatal insulin treatment. The birth size of infants born with
marked fetal hypoinsulinemia suggests that the human fetus can reach
the size of a 30 to 32 week gestation fetus independent of insulin.
The evidence suggests that insulin not only has a permissive role
but also a regulatory role in fetal growth96.
The insulin like growth factors.
The term somatomedin (SM) and insulin-like growth
factors(IGF) are synonymous. Somatomedin A and C are analogous to
IGF-I. IGF-II is equivalent to multiplication stimulating activity
(MSA), discovered in the rat. The IGFs share a large structural homology
with proinsulin.
IGFs do not cross the placenta and the IGF-I and the
IGF-II found in fetal plasma in various species are produced by fetal
tissues. Although in postnatal life the liver is the main producer
of IGF-I, during fetal life most tissues produce the same including
liver, heart, kidneys and skin103. Lung and intestine have
the highest concentration, liver the lowest. The concentrations in
these tissues are far in excess of amounts that can be accounted for
by contamination from the blood.
On the basis of these findings the classical concept
that IGFs act as endocrine factors has been progressively abandoned
in favour of the concept of paracrine or autocrine function. The concentrations
of IGFs in fetal plasma probably do not reflect the functional situation.
Most of the IGFs are bound to a carrier protein; total IGF concentration
does not reflect the hormone available to react with the receptors.
Fetal hormonal regulation differs from post natal life in the relative
role of growth hormone, which becomes apparent about 6 months postnatally
with a more autocrine and paracrine character of regulation96,97,104
IGF-I production is not controlled by GH. Fetal decapitation,
hypophysectomy, or electrocoagula 1000 tion of the hypothalamus of
the lamb or rat abolishes GH from the fetal circulation but has no
effect on circulating somatomedin or IGF-I levels. Moreover, GH fails
to stimulate IGF secretion by the rat fetus. or by cultured rat fibroblasts.
In addition, human anencephalic infants have normal somatomedin levels
in cord blood (reviewed in vol 18 p29). Insulin concentrations affect
IGF concentration. In situations where insulin levels are low, IGF-I
tends to be decreased whereas IGF-II remains normal. Conversely, in
situations of hyperinsulinaemia, IGF-I levels are increased. IGF-II
levels are unaffected.
Human placental lactogen (HPL) is a peptide secreted
by the placental trophoblast. HPL is secreted into the maternal and
the fetal circulations. HPL secreted in the maternal circulation stimulates
the mobilisation of metabolites and increases nutrient delivery to
the fetus, by acting as an insulin antagonist. Indirect evidence suggests
that it also stimulates maternal IGF-I secretion. HPL in the fetal
circulation stimulates IGF-I production. Based on the above findings,
the following working hypothesis has been proposed104:
fetal cellular growth is dependant on nutrient delivery (eg, glucose,
amino acids, and fatty acids). The uptake of nutrients by the fetal
cells is stimulated by insulin. Fetal insulin secretion is stimulated
by amino acids up to 28 weeks of gestation and by amino acids and
glucose thereafter. Cellular anabolism progresses to replication under
the influence of growth factors. Growth factor synthesis and release
are stimulated not only by intracellular events such as delivery of
fuel, which is augmented by insulin, but also by HPL, which may be
acting on many fetal tissues in a manner analogous to GH acting on
the hepatocyte postnatally. Finally, there is a long loop in which
insulin reinforces the anabolic process by stimulating HPL secretion.
Conclusions
There is a very intricate relationship between mother
and child which lasts beyond the birth of the child. The nutritional
status of the mother and her energy and protein intake during pregnancy
play an important role, but they explain only part of the observed
growth outcomes of the child. This could be due to the fact that the
timing of the insult is important and in most studies not taken into
consideration and because of the role micronutrients are likely to
play.
The role of micronutrients is difficult to interpret
when interaction between different elements at the biochemical level
is concerned. For some minerals the link between endowment at birth
in these elements and the subsequent growth of the child are clear.
The iron status and vitamin A status of the newborn are determined
by gestational age and the reserves the mother had. Food diversity
is particularly a determinant of the intake of micronutrients. This
could then perhaps explain why growth faltering is found to start
earlier in rural populations as compared to urban ones. The most likely
candidates to possibly affect linear growth of children are vitamin
A, zinc and iron, two of which belong to the most important micronutrient
deficiencies worldwide.
Perhaps it is time for a research reorientation more
towards the study of the relationship between fetal growth and early
postnatal linear growth. So far research has concentrated predominantly
on the relationship between macro-nutrients and birth outcome, this
mostly expressed as birth weight. But linear growth of children, particularly
early after birth could also be an indicator of what one could consider
the "hidden hunger". The nutritional status, more particularly
the micro-nutrient homeostasis of women at the time of conception,
might have important repercussions for young children.
1000 Chinese
abstract
References
- Child malnutrition. Progress toward the world summit
for children goal. New York: UNICEF, 1993.
- Karlberg J. A biologically-oriented mathematical
model (ICP) for human growth. Acta Pediatr Scand 1989; Suppl. 350:
70-94.
- Karlberg J, Albertsson-Wikland K. Infancy growth
pattern related to growth hormone deficiency. Acta Pediatr Scand
1988; 77: 385-391.
- Karlberg J, Jalil F, Lindblad S. Longitudinal analysis
of infantile growth in an urban area of Lahore, Pakistan. Acta Pediatr
Scand 1988;77:392-401.
- Karlberg J, Engström I, Karlberg P, Fryer JG. Analysis
of linear growth using a mathematical model I. From birth to three
years. Acta Pediatr Scand 1987;76:478-488.
- Karlberg J, Henter J, Tassin E. Longitudinal analysis
of infantile growth in children with coeliac disease. Acta Pediatr
Scand 1988;77:516-524.
- Waterlow JC. Causes and mechanisms of linear growth
retardation (stunting). Eur J Clin Nutr 1994;48 (Suppl.1): S1-S4.
- Waterlow JC. Reflections on stunting. Int Child
Health 1991; 2 No.2: 25-35.
- Golden MHN. Is complete catch-up possible for stunted
malnourished children? Eur J Clin Nutr 1994; 48 (Suppl.1): S58-S71.
- Kusin JA, Sri Kardjati, Houtkooper JM, Renqvist
UH. Energy supplementation during pregnancy and postnatal growth.
Lancet 1992; 340: 623-626.
- Harrison GA, Brush G, Zumrawi FY. Interrelations
between growth, weaning and disease experience in Khartoum infants.
Eur J Clin Nutr 1992; 46: 273-278.
- Gueri M, Gurney JM, Jutsum P. The Gomez classification.
Time for a change? Bull WHO 1980; 58: 773-777.
- Stewart AL, Reynolds EOR, Lipscomb AP. Outcome
of very low birthweight: survey of world literature. Lancet 1981;
1038-1041.
- Brimblecombe FS, Ashford JR, Fryer JG. Significance
of low birth weight in perinatal mortality. Brit J prev soc Med
1968; 22: 27-35.
- Mavalankar DV, Gray RH, Trivedi CR. Risk Factors
for preterm and term low birthweight in Ahmedabad India. Int J Epidemiol
1992; 21: 263-272.
- Cruise MO. A longitudinal study of the growth of
low birth weight infants. l. Velocity and distance growth, birth
to 3 years. Pediatr 1973; 51: 620-628.
- Westwood M, Kramer MS, Munz D, Lovett JM, Watters
GV. Growth and development of full-term non asphyxiated small-for-gestational-age
newborns: follow-up through adolescence. Pediatr 1983; 71: 376-382.
- Fitzhardinge PM, Stevens EM. The small for date
infant. I. Later growth patterns. Pediatr 1972; 49: 671-681.
- Martell M, Falkner F, Bertolini LB, et al. Early
postnatal growth evaluation in full-term, preterm and small for
dates infants. E Hum Dev 1978; 1/4: 313-323.
- Georgieff MK, Sasanow SR, Mammel MC, Pereira GR.
Mid-arm circumference/head circumference ratios for identification
of symptomatic LGA, SGA, and SGA newborn infants. J Pediatr 1986;
109: 316-321.
- Tenovuo A, Kero P, Piekkala P, Korvenranta H, Sil
1000 lanpää M, Erkkola R. Growth of 519 small from gestational age
infants during the first two years of life. Acta Pediatr Scand 1987;
76: 636-646.
- Kramer MS, McLean FH, Olivier M, Willis DM, Usher
RH. Body proportionality and head and length sparing
in growth retarded neonates: a critical reappraisal. Pediatr 1989;
84: 717-723.
- Waterlow JC. Note on the assessment and classification
of protein-energy malnutrition in children. Lancet 1973; 2: 87-89.
- Miller HC, Jekel JF. Malnutrition and growth retardation
in newborn infants. Pediatr 1989; 83: 443-444.
- Hill RM, Verniaud WM, Deter RL, et al. The effect
of intrauterine malnutrition on the term infant. A 14 year progressive
study. Acta Pediatr Scand 1984; 73: 482-487.
- Kramer MS, Olivier M, McLean FH, Dougherty GE,
Willis DM, Usher RH. Determinants of fetal growth and body proportion.
Pediatr 1990; 86: 18-26.
- Miller HC, Hassanein K. Diagnosis of impaired fetal
growth in newborn infants. Pediatr 1971; 48: 511-522.
- Falkner F, Holzgreve W, Schloo RH. Prenatal influences
on postnatal growth: overview and pointers for needed research.
Eur J Clin Nutr 1994; 48 (Suppl.1): S15-S24.
- Pomerance HH, Krall JM. The relationship of birth
size to the rate of growth in infancy and childhood. Am J Clin Nutr
1984; 39: 95-99.
- Garn SM, LaVelle M. Interaction between maternal
size and birth size and subsequent weight gain. Am J Clin Nutr 1984;
40: 1120-1121.
- Davies DP. The importance of genetic influences
on growth in early childhood with particular reference to children
of Asiatic origin. In: Waterlow JC, ed. Linear growth retardation
in less developed countries. Nestle Nutrition Workshop Series. Vol.14.
New York: Raven Press, 1988: 75-90.
- Smith DW, Truog W, Rogers JE, Reizer LJ, Skinner
AL, McCann JJ. Shifting linear growth during infancy: illustration
of genetic factors in growth from fetal life through infancy. J
Pediatr 1976; 89: 225-230.
- Habicht JP, Martorell R, Yarbrough C, Malina RM,
Klein RE. Height and weight standards for preschool children. How
relevant are ethnic differences in growth potential? Lancet 1974;
611-614.
- Rona RJ. Genetic and environmental factors in the
control of growth in childhood. Br Med Bull 1981; 37: 265-272.
- Roberts DF. Genetics of growth. Br Med Bull 1981;
37: 139-246.
- Marshall WA. Geographical and ethnic variations
in human growth. Br Med Bull 1981; 37: 273-279.
- Tanner JM. Population differences in body size,
shape, and growth rate. A 1976 view. Arch Dis Childh 1976; 51: 1-2.
- Martorell R, Mendoza F, Castillo R. Poverty and
stature in children. In: Waterlow JC, ed. Linear growth retardation
in less developed countries. Nestle Nutrition Workshop Series. Vol.14.
New York: Raven Press, 1988: 57-73.
- Castillo-Duran C, Uauy R. Copper deficiency impairs
growth of infants recovering from malnutrition. Am J Clin Nutr 1988;
47: 710-714.
- Kusin JA, Jansen AJ. Maternal nutrition and birthweight:
selective review and some results of observations in M 1000 achakos,
Kenya. Ann Trop Paediatr 1986; 6: 3-9.
- Robyn C, Keita MS, Meuris S. Intrauterine growth
retardation in Africa. In: Senterre J, ed. Intrauterine growth retardation.
Nestle Nutrition Workshop Series. Vol 18. new York: Raven Press,
1989: 165-181.
- Wharton B. Causes of low birthweight in developing
countries. In: Senterre J, ed. Intrauterine growth retardation.
Nestle Nutrition Workshop series. Vol 18. New York: Raven Press,
1989: 143-155.
- de Nobrega FJ, Lopez FA, Segre CAM. Anthropometry
in Brazilian newborn infants: Studies of association with some maternal
factors. In: Senterre J, ed. Intrauterine growth retardation. Nestlé
Nutrition Workshop Series. Vol 18. New York: Raven Press, 1989:
157-164.
- Bhargava V, Chatterjee M, Prakash A, Bhatia B,
Mishra A. Fetal growth variations. l. Influence of maternal size
and nutrition on identification of fetal growth retardation. Ind
Pediatr 1983; 20: 549-559.
- Rush D, Stein Z, Susser M. A randomized controlled
trial of prenatal nutritional supplementation in New York city.
Pediatr 1980;65:683-697.
- Osofsky HJ. Relationship between prenatal medical
and nutritional measures, pregnancy outcome and early infant development
in an urban poverty setting. Am J Obstet Gynecol 1975; 682-690.
- Viegas OAC, Scott PD, Cole TJ, Eaton P, Needham
PE, Wharton BA. Dietary protein energy supplementation of pregnant
Asian mothers at Sorrento, Birmingham. I. Unselective during second
and third trimester. Br Med J 1982; 285: 589-592.
- Viegas OAC, Scott PD, Cole TJ, Eaton P, Needham
PE, Wharton BA. Dietary protein energy supplementation of pregnant
Asian mothers in Sorrento, Birmingham. ll. Selection during the
third trimester only. Br Med J 1982; 285: 592-595.
- McDonald EC, Pollit E, Mueller W, Hsuek AM, Sherwin
R. the Bacon-Chow study: maternal nutritional supplementation and
birth weight of offspring. Am J Clin Nutr 1981; 34: 2133
- Lechtig A, Habicht JP, Delgado H, Klein RE, Yarbrough
C, Martorell R. Effect of food supplementation on birth weight.
Pediatr 1975; 508-520.
- Mora JO, de Paredes P, Wagner M, et al. Nutritional
supplementation and outcome of pregnancy. I. Birthweight. Am J Clin
Nutr 1979; 32: 455-462.
- Prentice AM, Whitehead RG, Watkinson M, Lamb WH,
Cole TJ. Prenatal dietary supplementation of African women and birthweight.
Lancet 1983; i: 489-492.
- Susser M. Maternal weight gain, infant birth weight,
and diet: causal sequences. Am J Clin Nutr 1991; 53: 1384-1396.
- Suescun J, Mora JO. Food supplements during pregnancy.
In: Senterre J, ed. Intrauterine growth retardation. Nestle Nutrition
Workshop Series Volume 18. New York: Raven Press, 1989: 223-241.
- Mardonnes-Santander F, Rosso P, Stekel A, et al.
Effect of a milk-based food supplement on maternal nutritional status
and fetal growth in underweight Chilean women. Am J Clin Nutr 1988;
47: 413-419.
- Adair LS, Pollit E. Outcome of maternal nutritional
supplementation: a comprehensive review of the Bacon-Chow study.
Am J Clin Nutr 1985;41 :948-978.
- Moser-Veillon PB, Reynolds RD. A longitudinal study
o