Asia Pacific J Clin Nutr (1995) 4: 47-50

Can total body nitrogen be measured
in newborn infants?
DJ Borovnicar1 and DB Stroud2
- Department of Medicine, Monash University, Clayton
3168;
- Department of Medical Physics, Monash Medical
Centre, Clayton 3168, Australia.
The feasibility of using prompt in vivo neutron
activation Analysis (IVNAA) of nitrogen to measure the total body
nitrogen (TBN) of newborn infants has been investigated by redesigning
and recalibrating an existing IVNAA facility used for the measurement
of TBN in adults. Repeated 1000 sec measurements of an infant phantom
(4kg wt: 80g N) yielded an average measured value that is within
0.2± 1.8% (1xSD) of the actual value
and a precision of 7.9% (CV) for a single measurement. Preliminary
investigations indicate that the whole body radiation dose is no
greater than 1 mSv (Q=20) for a 1000 s irradiation. It is proposed,
and in part demonstrated, that measurement precision can be reduced
to » 5% by (i) using a graphite
neutron reflector positioned over the infant to increase the in
vivo thermal neutron flux, and (ii) doubling the number of NaI(TI)
detectors.
Introduction
There is increasing evidence to suggest that total
body nitrogen (TBN) is an important indicator of nutritional status
in health and disease1, nitrogen being related to protein
by a relatively fixed ratio of 1:6.25. Although several clinical centres
have established non invasive methods of measuring TBN in adults2-5
and children6-7, no method has been routinely applied to
measure the TBN of newborn infants8. If this could be achieved,
it would contribute significantly to the early diagnosis and effective
management of diseases affecting protein stores during the most formative
period of growth, increasing the likelihood of a favourable prognosis.
At present in vivo neutron activation analysis (IVNAA)
is the only non-invasive method for the in vivo measurement of TBN.
In the prompt g -IVNAA method the subject is irradiated
with fast neutrons which are subsequently moderated within the patient
and then captured by nitrogen nuclei according to the reaction 14N(n,
g )15N. The prompt y-ray released
in this reaction has a characteristic energy of 10.83 MeV and can
be identified and counted by NaI detectors. The number measured over
a 1000 s period will be referred to as the signal (S) where it is
assumed that S is proportional to the mass of nitrogen irradiated.
The gross signal coming from the detectors contains a background (B)
component which must be measured separately and then subtracted to
obtain S.
This method is routinely applied to adults at the
Monash Medical Centre (MMC). In particular, a collimated neutron beam
(20 cm x 40 cm at bed level) from a 10 m g 252Cf source (2nd
July, 1992) unilaterally irradiates the trunk section of a static
subject for 1000 s whilst two 10 cm x 10 cm x 15 cm NaI(TI) detectors
positioned bilaterally 50 cm apart measure S with a precision of » 4% from an effective radiation dose of 0.4 mSv (Q=20)9.
If this method for adults were applied to infants,
the measurement precision would be unsatisfactory. To illustrate,
consider the standard man weighing 70 kg and containing 1800 g of
nitrogen10. Only half of this nitrogen mass, ie 900 g,
is irradiated. The gross counts from an adult measurement is 2800
with a background of approximately 900 per 1000 s. However, an infant
is assumed to weigh 4 kg,80 g of which is nitrogen10. Hence
the nitrogen counts from an infant would be reduced by a factor of
ten because the quantity of nitrogen being irradiated is approximately
one-tenth of that in the adult (ie 80 g compared with 900 g). For
an infant measurement, B would remain at about 900 counts but the
gross counts would be 990 (ie 900 + 0.1 [2800-900]) which would give
a measurement precision11 of 48% for S. This could be improved
by counting for a longer period of time, but this would increase the
radiation dose to the infant.
In view of these difficulties, a study was conducted
to determine whether the MMC IVNAA facility could be redesigned and
calibrated to measure the TBN of infants with a precision of » 5% and a radiation dose comparable to that for adults. This study follows
the work of Wang et al.8 which considered the measurement
of various small animals. Although no infants were measured, these
works demonstrate the potential for the measurement criteria of this
study to be met.
Design study of the IVNAA facility
Figure 1 is a cross-sectional view of the infant IVNAA
facility. The design aims were to (1) maximize S, which is proportional
to the in vivo thermal neutron flux and the detection efficiency for
10.83 MeV g -rays, and (2) to minimize B. Signal was
optimized by investigating: (I) the neutron collimator composition
and geometry, (2) the number and positioning of NaI(TI) detectors,
(3) the use of a fast neutron pre-moderator inserted between the neutron
source and the subject, and (4) the use of graphite positioned above
the subject to reflect leaked neutrons back into the subject. Background
was reduced by investigating: (1) neutron collimator geometry and
(2) neutron and g -ray shielding composition and geometry.
Figure 1.
Cross sectional view of the IVNAA facility for the measurement
of TBN in newborn infants. |
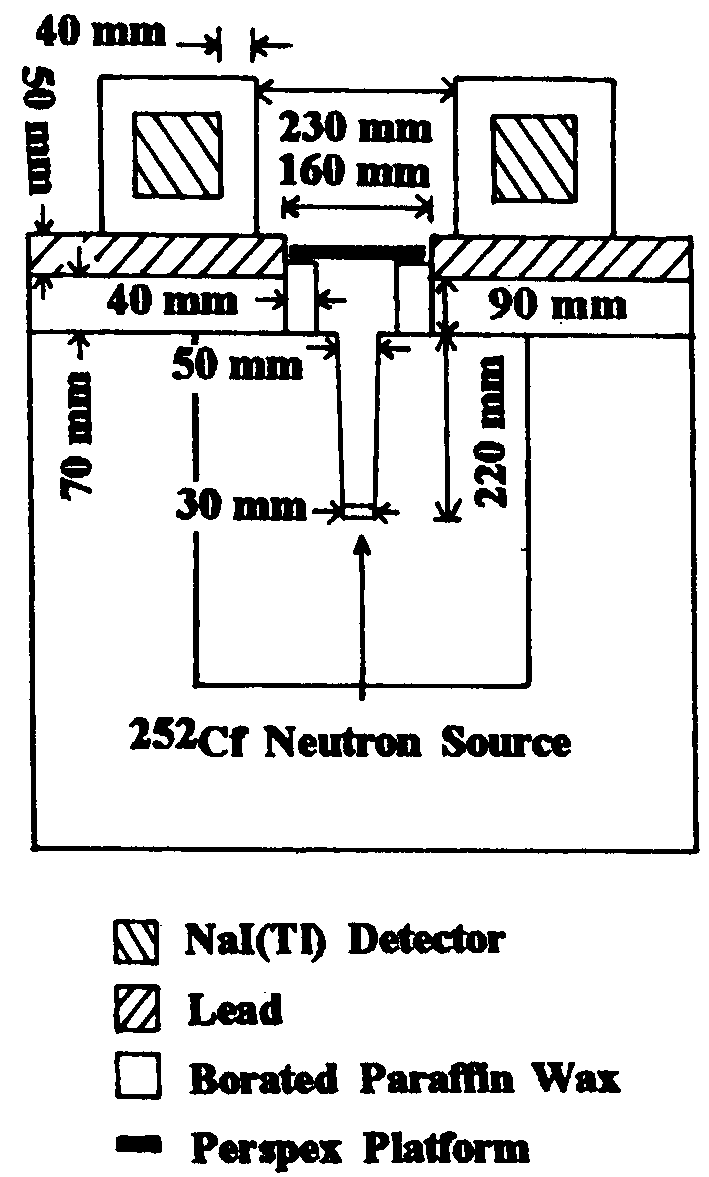 |
Signal maximization
The 252Cf source is positioned at the apex
of an inverted rectangular conical void cast within a paraffin wax
block (40 cm x 40 cm x 60 cm) doped with boric acid (H3BO3,
» 5% wt). The size of the neutron beam exiting the collimator is defined
by an aperture measuring 5 cm x 30 cm. A narrow aperture width increases
the proportion of slow neutrons in the neutron beam and reduces the
dose to the patient12,13 whilst the aperture length ensures
maximum exposure along the subject. A narrow neutron beam also makes
possible the reduction in separation of the NaI detectors from 50
to 25 cm without substantially increasing B; this increases the geometrical
detector efficiency by a factor of four according to the inverse square
law. Two additional NaI(TI) detectors of equal volume are awaiting
installation; this will increase S by a further factor of two.
The effects of a pre-moderator
were studied by inserting a 2 cm thick paraffin wax block between
the 252Cf source and subject and performing 1000 s
counts on calibration phantoms. Results are shown in Table 1.
It was expected that this would increase the thermal neutron flux
within the nitrogen phantom and hence increase S. The experiment
resulted in a significant reduction in S (» 11%) and a somewhat less
significant increase in B, demonstrating that the effect of a
premoderator can be quite complex (see Table 1).
Unilateral irradiation results in a significant
proportion of neutrons passing through the subject. In a final
set of measurements, a graphite slab was placed above the phantom
to determine if these neutrons could be reflected back into
the phantom and increase S relative to B. This was tested using
a 15 cm x 5 cm x 40 cm graphite slab positioned » 5 cm directly over the infant phantom. Results are shown in
Table 2 for a 1000 s irradiation. A statistically significant
increase in S was noted, leading to an improved precision. Stamatelatos14
has investigated this effect in some detail and reported results
that support this observation.
|
Table 1. Effect of a 2 cm
wax premoderator on the measured value of S.
|
Signal (s) |
Background (B) |
No wax premoderator
|
1544± 54 |
713± 27 |
Wax premoderator
|
1372± 54 |
763± 27 |
Table 2. Effect of a graphite reflector
positioned above the infant phantom.
|
No Graphite Reflector |
Graphite Reflector |
Signal (S) + statistical
error (s ) |
474± 36 |
639± 38 |
Background (B)
+ statistical error (s ) |
790± 28 |
808± 28 |
s /S x 100% |
9.6% |
7.4% |
|
Background minimization
The major background component of a g -ray spectrum (B) arises from the
interaction of neutrons and g -rays with the NaI(TI) crystal11
. Although detection of B is unavoidable, the geometry and composition
of shielding materials have been designed to minimize B.
Borated paraffin wax above the neutron collimator
moderates and captures leaked neutrons and a 5 cm thick lead shield
absorbs Y and neutron radiation. The narrow collimator directs the
neutron beam away from the detectors and onto the subject.
Calibration of TBN measurement
Body hydrogen is used as an internal standard when
measuring nitrogen15; this is implemented by counting the
prolific 2.22 MeV g -rays arising from neutron capture in body
hydrogen. Subject measurements are calibrated against a phantom of
similar shape and size containing a known mass of hydrogen and nitrogen.
The background in the 10.83 MeV region of the g -ray spectrum (nitrogen background [NB]) is determined using a plain
water phantom. Background in the 2.22 MeV region (hydrogen background
[HB]) is determined with no phantom positioned above the neutron source
(ie 'air phantom').
In particular, it is
difficult to determine HB. This is because a significant proportion
of HB arises from the subject reflecting neutrons into the collimator
and shielding materials where 1H nuclei are subsequently
activated, this effect varies with subject size16.
Table 3 presents results of measurements that have been performed
on two different phantoms to determine HB. The results demonstrate
that HB has the potential to significantly affect the determination
of the subject's hydrogen count rate because HB is so large. However,
the observed 15% difference in HB translates into a systematic
error of only 1.1% when calculating TBN. This insensitivity arises
because TBN depends on the ratio of two hydrogen count rates (phantom/
subject) and this ratio is less sensitive to the value of HB.
Although this does not dismiss the need to carefully quantify
the effect of varying subject dimensions, for the purpose of this
study, air phantom measurements with a +l5% correction were used
to estimate HB.
Results of accuracy and precision measurements
Methodological accuracy and precision were determined
from 20 1000 s exposures of a 4 kg infant phantom (16 cm x 8
cm x 38 cm) filled with urea solution containing 80 g of nitrogen.
The precision of measuring a 3 kg sample of minced Re beef was
also determined8. Every measurement was independently
calibrated against a calibration phantom where no graphite reflector
was used. Table 4 details the results.
|
Table 3. Measured estimates
of the hydrogen background (HB).
|
Infant phantom(4 kg Urea solution:80g N) |
Air phantom Graphite phantom (15x5x40 cm3) |
|
Total H counts
(S+B) |
1 962 725 |
|
|
Measured 1HB |
|
701 226 |
799 150 |
Measured 1HB
as % of total 1H counts of IPa |
|
35.7% |
40.7% |
aInfant phantom
Table 4. Nitrogen measurements of an
infant phantom and a sample of minced beef.
Phantom type |
Mass (g) |
No. of independent measurements |
Actual N mass (g) |
Measured N mass(g) |
CV |
Urea solution
|
4000 |
20 |
80.0± 0.2 |
80.1± 6.4 |
7.9% |
Minced beef |
3000 |
7 |
unknown |
92.4± 3.7 |
4.0% |
|
The actual nitrogen content of the infant phantom
is within 0.2% of the average measured value and within the associated
error range predicted by nuclear statistics (± 1.8% SD); this suggests the accuracy
of the method to be better than » 98%. Reproducibility measurements
of the infant phantom suggest the precision of the method to be 7.9%
(CV); this agrees with the » 10% value predicted by nuclear
statistics.
The measured nitrogen content of the beef sample is
consistent with similar measurements performed elsewhere8
and with the expectation that an increased nitrogen content results
in an increased measurement precision.
Dosimetry
The radiation dose to the subject arises from neutrons
and g -radiation, fast neutrons being the major dose component13.
The dose equivalent was measured at both the anterior and posterior
surface of the infant phantom using standard personnel radiation dosimeters17.
A conservative calculation of the effective dose equivalent to the
whole body was then performed with the infant assumed Iying supine.
This preliminaly investigation yielded an upper limit of 1 mSv (Q=20);
no graphite reflector was considered. This value compares to a newbom
infant chest X-ray effective dose equivalent of 0.1 mSv. Dose measurements
have not yet been performed with the graphite reflector in position.
Discussion
This study demonstrates that prompt IVNAA can be used
to measure the TBN of newborn infants (» 4 kg mass) with a precision of
7.9% and an accuracy better than 98% for an effective dose equivalent
value to the whole body no greater than 1 mSv (Q=20). If the number
of detectors are doubled and a graphite neutron reflector positioned
above the subject, the measurement precision is expected to decrease
from » 10% to » 5% making the method useful in
clinical applications.
Further work is required to quantify the calibration
procedure for subjects of varying dimensions. More importantly, an
accurate measurement and calculation of the radiation dose is required
to establish the level of health risk to which the subject is exposed.
However, it is encouraging to note that the effective dose equivalent
estimated in this study is at most 1 mSv. This order of exposure has
been classed by the ICRP to represent a minor level of health risk
and would be justified if the experiment produces a health benefit18.
Acknowledgments - The support and collaboration
of A.H. Beddoe during the initial stages of this study is gratefully
acknowledged. Special thanks are extended to Prof M.L. Wahlqvist and
to Dr B.J.G. Strauss for his enthusiastic support. DJB is funded by
a Monash University Departmental Scholarship.
References
- Allen BJ, Pollock CA, Russell J, Oliver C, Smith
R. Role of body protein as a prognostic indicator in wasting disease.
Asia PacificJClinNutrl995; 1:31-33.
- McNeill KG, Mernagh JR, Jeejeebhoy KN, Wolman SL,
Harrison JE. In vivo measurements of body protein based on the determination
of nitrogen by prompt y analysis. Am J Clin Nutr 1979; 32: 1955-1961.
- Beddoe AH, Zuidmeer H and Hill GL. A prompt gamma
in vivo neutron activation analysis facility for measurement of
total body nitrogen in the critically ill. Phys Med Biol 1984; 29:
371 -383.
- Ryde SJS, Morgan WD, Evans CJ, Sivyer A, Dutton
J Calibration and evaluation of a 252Cf-based neutron activation
analysis instrument for the determination of nitrogen in vivo. Phys
Med Biol 1989;34: 1429-1441.
- Vartsky D, Ellis KJ, Cohn SH. In vivo measurement
of body nitrogen by analysis of prompt gammas from neutron capture.
J Nucl Med 1979;20: 1158-1165.
- Baur LA, Allen BJ, Rose A, Blagojevic N, Gaskin
KJ. A total body nitrogen facility for pediatric use. Phys Med Biol
1991; 36: 1363-1375.
- Ellis JE, Shypailo RJ. Nuclear based techniques
for the measurement of body composition: applications in pediatric
research. In: Kral JG, Vanitallie TB, eds Recent developments in
body composition analysis: methods and applications. Smith-Gordon,
1993: 99-110.
- Wang H, Waana C, McNeill KG, Harrison JE, Tbompson
L. In vivo measurement of nitrogen for small animals using nuclear
techniques. Phys Med Biol 1987; 32: 637-641.
- Stroud DB, Borovnicar DJ, Lambert JR, McNeill KG,
Marks SJ, Rassool RP, Rayner HC, Strauss BJG, Tai EH, Thompson MN,
Wahlqvist ML, Watson BA, Wright CM. Clinical Studies of Total Body
Nitrogen in an Australian Hospital. In: Yasumura S, Harrison JE,
McNeill KG, Woodhead AD, Dilmanian FA, eds In vivo body composition
studies: recent advances, Plenum Press,1990: 177-182.
- Forbes BG. Human body composition. Growth, aging,
nutntion andactivity.Springer-Verlag,1987.
- McNeill KG, Borovnicar DJ, Krishman SS, Haiying
W, Waana Ct Harrison JE. Investigation of factors which lead to
the background in the measurement of nitrogen by IVNAA. Phys Med
Biol 1989; 34: 53-59.
- Mountford PJ. Effect of collimator and depth in
a phantom on the neutron spectrum from a 23sPu,Be neutron source.
Phys MedBiol 1982;27: 1245-1252.
- McGregor BJ, Allen sJ. Monte carlo study of neutron
and gamma ray transport in the AAEC total body nitrogen facility.
Aust Phys Eng Sci Med 1987; 10: 155-161.
- Stamatelatos IAE, Chettle DR, Green S, Scott MC.
Design studies related to an in vivo neutron activation analysis
facility for measuring total body nitrogen. Phys Med Biol 1992;
37: 1657-1674.
- Vartsky D, Prestwich WV, Thomas BJ, Dabek JT, Chettle
DR, Fremlin JH, Stammers K. The use of body hydrogen as an internal
standard in the measurement of nitrogen in vivo by prompt 18 neutron
capture gamma-ray analysis. J Rad Chem 1979; 48: 243-252.
- Humphries IRJ, Allen BJ, Gaskin KJ. Hydrogen background
in total body nitrogen (TBN) estimations. Private Communi cation,
1993.
- Morris ND. Personal radiation monitoring and assessment
of doses received by radiation workers. Australian Radiation Laboratory
I 991; ARL/TR I 07.
- Annals of the ICRP. Radiological protection in
biomedical research. Pergamon Press, 1991; ICRP publication 62.

Copyright © 1995 [Asia Pacific Journal of Clinical
Nutrition]. All rights reserved.
Revised:
January 19, 1999
.